Building for 2050: Low cost, low carbon homes
Updated 5 December 2022
Acknowledgements
BEIS would like to thank the research team, steering group members, case study housing developers, design and construction teams, and residents; survey participants, webinar attendees, and all other stakeholders who contributed to the production of this report.
Glossary
- Airtightness: High levels of airtightness reduce heat loss as there is less uncontrolled air movement in and out of a building.
- Ambient loop: A communal heating system with an ambient loop is one in which the hot water is distributed at a lower temperature than is traditional. This approach is suited to lower temperature heating sources such as heat pumps, and it reduces system losses and the risk of overheating in corridors.
- ASHP: An Air Source Heat Pump is a low carbon heating system that compresses outside air to a higher temperature to heat rooms via radiators or underfloor heating. It can also heat water stored in a hot water cylinder.
- Capex: An abbreviation of capital expenditure.
- Carbon emissions: A term used to refer to both carbon dioxide (CO₂) emissions released into the atmosphere from burning fossil fuels (coal, oil, natural gas) and other greenhouse gas emissions. The full term is carbon dioxide equivalent emissions (CO₂e).
- Carbon intensity: The carbon intensity of electricity is the number of grams of carbon dioxide that it takes to make one unit of electricity (a kilowatt) per hour (gCO₂/kWh).
- Climate Change Act: The Climate Change Act commits the UK government by law to reducing greenhouse gas emissions by at least 100% of 1990 levels (net zero) by 2050.
- CLT: Cross-laminated Timber is a wood panel product made from gluing together layers of lumber.
- Code for Sustainable Homes: A now-retired, national environmental assessment method for rating and certifying the performance of new homes, ranging from level 1 to level 6 (best performance). With level 6, the home is at least zero carbon (zero net emissions of carbon dioxide from all energy use in the home).
- D&B: Design and Build. A contract type for which the building contractor is responsible for design.
- Decarbonisation: The reduction of carbon. In the context of grid decarbonisation, it relates to the reduction of carbon dioxide emissions through the use of low carbon sources to generate electricity.
- DHW: An abbreviation of Domestic Hot Water.
- DNO: Distribution Network Operators manage the electric power distribution system which delivers electricity to most end users.
- EEB: An Earth Energy Bank stores heat in earth underground, which is used to heat buildings.
- Energy positive/plus: Energy positive (or plus) buildings generate more energy than they consume, for example using solar technology.
- EPC: An Energy Performance Certificate summarises the energy efficiency of a building, using a rating of A to G (A is the best performance).
- ESG: A set of corporate standards that measure a company’s sustainability in 3 specific categories: environmental, social and governance.
- Fabric-first: A design and construction strategy that focusses initially on reducing the heat loss through the building fabric.
- Future Homes Standard: The Future Homes Standard is the Part L standard that is to apply to new homes built from 2025. It is expected to be future-proofed through requiring low carbon heating and a high standard of energy efficiency.
- GSHP: A Ground Source Heat Pump is a low carbon heating system that extracts low-temperature energy stored in the ground or water, using buried pipework, and compresses this energy into a higher temperature to heat buildings.
- Heat and Buildings Strategy: The government strategy setting out how the UK will decarbonise homes, and other buildings, as part of setting a path to net zero by 2050.
- HIU: A Heat Interface Unit delivers heat to an individual dwelling from a centralised heating plant or district heating scheme.
- M&E: The mechanical and electrical services in a building.
- MEP consultant: Provider of mechanical, electrical, and plumbing consultancy services.
- MEV: Mechanical Extract Ventilation - mechanically driven ventilation that continuously extracts indoor air and discharges it to outside.
- MVHR: Mechanical Ventilation with Heat Recovery - mechanically driven ventilation that both continuously supplies outdoor air to the inside of the dwelling and continuously extracts indoor air and discharges it to the outside. In doing so, it retains most of the energy that has already been used in heating the building.
- MMC: Modern Methods of Construction - the design, planning, manufacture and pre-assembly of construction elements or components in a factory environment, prior to installation on site at their intended, final location.
- Modular SIP system: Structural Insulated Panels are a high-performance, prefabricated building system consisting of an insulating foam core sandwiched between 2 structural facings.
- Net zero: When the UK’s total greenhouse gas emissions are equal to or less than the emissions the UK removes from the environment.
- Net Zero Strategy: Introduced in October 2021, this government strategy sets out policies and proposals for decarbonising all sectors of the UK economy to meet a net zero target by 2050.
- Operational carbon dioxide: The emissions of carbon dioxide and other global warming gases during the in-use operation of a building; this is distinct from whole life carbon (below).
- Part L: Focused on the conservation of fuel and power, this Building Regulation sets the standard for the energy performance of new and existing buildings.
- Part O: Focused on overheating, this Building Regulation is concerned with the design and construction of residential buildings that limit solar gain during the summer months, and provide occupants with adequate means of removing excess heat from indoor environments.
- Part S: This Building Regulation is concerned with future-proofing buildings and infrastructure, to be ready for electric vehicles.
- Passivhaus: Passivhaus is a quality assured, performance-based set of design criteria and a methodology for very low energy buildings. The aim is to eliminate the need for space heating and cooling, based on the principle that reducing heating loss to a minimum is the most cost-effective and most robust way of achieving a low carbon building. The key design features include a simple compact shape, optimisation of passive solar gain, super insulation and minimal thermal bridging, stringent levels of airtightness and mechanical ventilation with heat recovery.
- POE: Post Occupancy Evaluation is the process of obtaining feedback on a building’s performance in use after it has been built and occupied.
- PV: Solar photovoltaic (PV) panels convert sunlight into electricity.
- PV-T: PV-Thermal panels convert sunlight into both electricity and hot water.
- Renewable energy: Energy from a source that is not depleted when used, such as wind or solar power.
- Retrofit: The process of augmenting an existing building with new parts or equipment, to improve its functionality and performance.
- RIBA: Royal Institute of British Architects.
- RIBA plan of work: A framework that organises the process of briefing, designing, delivering, maintaining, operating, and using a building into 8 distinct stages.
- SAP calculations: Standard Assessment Procedure calculations assess and compare the energy and environmental performance of dwellings.
- SHEM: Smart Home Energy Management is a residential demand response tool that shifts and curtails demand to improve the energy efficiency and reduce electricity cost based on the real-time electricity price and user comfort. <
- Sustainable design: An environmentally responsible and resource-efficient building design approach encompassing, construction, maintenance, renovation, and reuse.
- SCoP: Seasonal Coefficient of Performance is a measurement that shows how effective a heat pump is on an annual basis and makes it easier to compare heat pumps across producers (and models from the same manufacturer).
- SMEs: Small and Medium-sized Enterprises.
- Time-of-use tariff: A Time-of-use energy tariff has a different price for a unit of energy at different times of the day. It is intended to encourage customers to use energy at off-peak times when nationally energy consumption is lower.
- TRV: A Thermostatic Radiator Valve automatically controls the temperature in a space by limiting the amount of hot water entering the radiator.
- TSC: A Transpired Solar Collector uses the power of solar radiation to pre-heat the air for a building’s heating system.
- Tyneside flats: Usually a single storey flat within a two-storey terraced house.
- Whole life carbon: The carbon emissions resulting from the materials, construction, and the use of a building over its entire life. This includes, if applicable, its demolition.
Executive summary
Building for 2050
Building for 2050 offers a snapshot of the UK’s construction industry and its ability to deliver low carbon homes now[footnote 1]. It identifies how best to minimise cost, improve energy efficiency, reduce carbon emissions, increase consumer demand, and accelerate industry delivery of low carbon housing. While the focus is new build housing in England and Wales, the key findings broadly apply to all new build housing throughout the UK.
Building for 2050 is unique research. In a detailed analysis of case study projects, it identifies the barriers and opportunities to their delivery at scale and examines the impact this could have on energy demand and carbon emissions.
It provides rounded analysis of the whole process from inception to occupancy - developing, designing, constructing, and living in low cost, low carbon homes. This holistic evaluation incorporates the views of multiple stakeholders and draws upon case study homes, the wider construction industry, and the energy services sector. A wide-ranging consumer survey and a general industry survey further contributed to the findings.
If all homes constructed in future are built to a low carbon standard, there should be no need to retrofit these homes before the UK government’s net zero 2050 deadline.
The project was led by AECOM and supported by Pollard Thomas Edwards (PTE), Four Walls, and LCP Delta (incorporating Delta-EE).
Methodology
This 5-year project – begun in 2017 and completed in 2022 – investigated low carbon housing to understand why and how this type of home is being built, how the residents use energy, and what their experiences are. It also set out to understand how the construction industry can deliver low cost, low carbon homes on a large scale, and asks - what are the drivers and barriers shaping this emerging sector?
The report is informed by literature and market reviews, 4 in-depth case studies of low carbon housing schemes across England and Wales, and surveys and interviews with technical and consumer audiences.
The case studies focussed on:
- Interviews with development teams on the delivery of low carbon homes
- Interviews with residents sharing their expectations and experiences with living in their homes. This covered energy bills, comfort, and the usability of low carbon technology.
- A design review of homes and site observations during construction and post-completion on the buildability of the designs;
- An assessment of additional low carbon capital costs;
- Post-completion testing of the thermal performance of building envelopes and mechanical ventilation systems;
- A comparison of the SAP (Standard Assessment Procedure) modelling undertaken by the development teams and by the research team;
- Monitoring of the energy and environmental performance of homes in use;
- Consideration of the operation of the homes in a changing energy system.
Today, no major housebuilder is building low carbon homes at scale. For this reason, the case studies focus on low carbon projects undertaken by small- and medium-sized developers. The 4 developers had different funding models and approaches to low carbon design. These factors, combined with the mix of tenures, provided a good range of research data.
Marmalade Lane Cohousing, Cambridge provides 42 dwellings, a mix of flats and houses, with a shared ‘Common House’ facility.
Active Homes, Neath, Wales provides 16 homes, a mix of houses and flats for social rent.
Etopia Homes, Corby, Northamptonshire provides 47 homes, flats and houses, for private sale.
Tallack Road, Waltham Forest, London is a private development providing a mix of 80% private and 20% affordable homes in 48 flats and 2 houses.
Low carbon homes: what did industry say?
What is enabling and driving low cost, low carbon homes?
- An understanding of the need for a step change in low carbon performance, in part to address fuel poverty concerns;
- A local vision for low carbon homes and an increased general awareness of the climate emergency;
- A business vision - developing the brand and products to address low or zero carbon targets;
- The chance to develop the local supply chain and benefit the local economy;
- Increased demand for real estate investments that meet Environmental, Social and Governance (ESG) funding criteria;
- Available sites in areas where local planning authorities have set ambitious carbon targets to respond to local conditions such as infrastructure capacity, land values or climate change concerns;
- Grant funding for low carbon technologies or advice;
- Investment by manufacturers in low carbon technologies;
- Collaboration with low carbon stakeholders. In some cases, expert advisors, either organisations or individuals were driving the low carbon homes.
What are the challenges and barriers?
- Achieving low cost homes, whilst embracing new technologies;
- Delivering lower running costs and improved comfort as well as low carbon homes;
- A lack of certainty about future requirements, and a perception of increased capital costs and challenging market conditions. Housebuilders said this is why they don’t build low carbon homes;
- A reluctance within industry to change supply chains and improve designs;
- A mismatch of knowledge and skills;
- Underdeveloped energy infrastructure;
- Concerns about negative consumer attitudes to innovative technologies and homes.
Living with low carbon homes: what did residents say?
What are the drivers?
The main factors influencing home buyers and renters are location, size, design, layout, and price. Specific drivers for those seeking low carbon homes included:
- Lower energy bills;
- A reduced environmental impact;
- Warmth, comfort and good air quality;
- New, modern and high-quality homes;
- Gas-free homes (considered to be safer as well as greener).
What are the barriers?
- More than 60% (of residents and potential residents) cited cost as a main barrier;
- A lack of availability of low carbon homes where people want to live;
- A lack of awareness of low carbon homes – it is not easy to identify these homes or their features when buying or renting homes;
- Concerns around performance, reliability and maintenance of the technologies used;
- The appearance of technologies and how much space they might take up;
- Fear of snagging issues and completion date slippage.
The pros and cons of living in low carbon homes
Case study residents had an expectation of lower energy bills, although for many of them, this wasn’t the case in practice. A focus on running costs at the design stage, design simplification and better co-ordination, greater on-site experience, and improved handover processes will help to mitigate this.
There were other benefits however: residents reported warm and comfortable internal environments and good air quality and ventilation in their low carbon homes.
Around half felt their homes imposed constraints on their behaviour. Getting to grips with technology, learning how to optimise controls, and then adapting how you live to suit, takes time. Some residents had to manage a daily routine around reduced availability of hot water. Early consideration of the residents, focusing on the daily operation of homes, and informed advice will help to address this in future homes.
Findings and next steps
The findings
The research has been distilled into 29 key findings, see Table 7, which lead to the key learning points.
Around half of the findings relate to increasing the supply and demand for low cost, low carbon homes, through consideration of the drivers and barriers for housebuilders and the wider industry, and highlighting the drivers and challenges for prospective and actual residents. The remaining points are focused on delivering low energy and carbon performance. Updated Building Regulations will drive the requirement for lower carbon homes. However, there is a risk that homes built to updated regulations will not perform in use as intended. Developers, design teams, and site-based teams need to understand why and how to ensure as-designed performance is delivered; this includes placing a greater emphasis on the end users in early thinking and on the handover process at the end. Knowledge-sharing is also key.
Achieving the UK’s Net Zero Carbon commitment by 2050, and the interim 2035 target, will require a focus wider than predicted carbon emissions from regulated operational energy. The research suggests this should include embodied carbon, the assessment of performance in-use, a recognition of the dynamic carbon intensity of grid-supplied electricity, and energy infrastructure upgrades to address the demands of all-electric new homes.
Next steps: Key learning points
Building for 2050 has identified 7 key learning points focused on increasing supply and demand of low energy and low carbon homes.
- Industry groups are requesting certainty on future requirements for low carbon homes - the scope and timing of regulatory requirements and clarity on the role of planning policy;
- Local planning policy is a key driver for lower carbon homes;
- The public sector can play a significant role in promoting more low carbon homes by setting clear performance requirements;
- In well-planned, well-constructed low carbon developments, the capital cost uplift is minimal and not a deterrent to delivery. Wider uptake would likely reduce this uplift. Highly complex designs aiming to deliver zero carbon or energy positive homes are likely to have on-costs considerably more than the market will pay for; grant funding was received by the 2 schemes with these more ambitious aims;
- Counter to consumer perceptions, the case study homes were no more costly to buy than standard homes (based on the low carbon features), but the broader issues of insufficient low carbon homes and easy-to-access information about them remain;
- There is likely to be an increased demand for low carbon homes if developers market them with details of features and running costs, and provide post-occupancy support to ensure they perform as promised;
- The construction sector and the energy services sector need to engage better. This will support delivery of low carbon homes, while avoiding significant energy infrastructure costs.
Seven further learning points have been identified, focused on the energy and carbon performance of low carbon homes.
- Performance in use should be improved with the adoption of simpler designs, which are easier to build and commission, and easier for residents to operate in accordance with the design intent;
- Industry should undertake further product development and housebuilders need to improve their knowledge of existing and emerging products and services;
- Developers and design teams should adopt a range of output metrics, not only low carbon targets;
- It is essential to ensure planned performance is delivered so that both running costs and carbon emissions are low;
- Developers need to focus on tailoring and resourcing the handover process for low carbon homes, so residents understand and operate their homes in an efficient manner;
- The interaction between dynamic energy use and the energy system should be better recognised in the Building Regulations;
- There is a need to upskill and improve collaboration across the industry.
Part 1: Introduction to the project and its findings
Part 1 of the report comprises the following Sections:
- Section 1 introduces the project;
- Section 2 explains the background and context;
- Section 3 details the approach taken;
- Section 4 presents headline findings from the case studies.
1. Introduction
By 2050 the UK needs to bring all greenhouse gas emissions to net zero. Homes – both new and existing – account for 17% of greenhouse gas emissions in the UK[footnote 2]. While considerable progress has been made, more must be done to decarbonise homes to help to meet the government’s commitment to achieve net zero greenhouse gas emissions across the UK by 2050.
BEIS commissioned the Building for 2050 research project to understand the drivers and barriers to large-scale construction and take-up of low cost, low carbon housing. The 5-year project was led by AECOM and supported by Pollard Thomas Edwards (PTE), Four Walls, and LCP Delta (incorporating Delta-EE).
The research team was tasked with identifying key lessons to minimise cost premiums, improve energy efficiency, reduce carbon emissions, increase consumer demand, and accelerate industry delivery. If all homes constructed in future are built to a low carbon standard, there should be no need to retrofit these homes before 2050. While the focus of the project is new build housing in England and Wales, the key findings are expected to broadly apply to new build housing across the UK. The project also provides useful insight for meeting the challenge of retrofitting existing homes at scale.
Building for 2050 has investigated new low carbon housing from inception to occupancy. A detailed evaluation was undertaken of 4 low carbon new build housing schemes from inception to occupancy. These case studies comprised interviews with the housing industry on the drivers, challenges, and experience of delivering low carbon homes, and feedback from residents on their expectations versus the reality of living in these homes. This was complemented by site observations during construction on the buildability of low carbon designs, performance testing of the homes at completion, and monitoring the energy and environmental performance of the homes during occupation. The report also captured wider perspectives from both industry and consumers. The original 3-year programme was extended to account for delays in the case study construction timetable, partly due to the COVID-19 pandemic.
This is unique research: it provides a holistic evaluation, drawing on findings from the case studies, and broader stakeholder engagement, including liaison with the energy services sector.
Developers were invited to propose case study developments, which were reviewed by BEIS and the research team. BEIS defined low cost, low carbon homes as low carbon homes which do not cost significantly more than standard new homes currently available on the market. The working definition used was homes in line with the following standards: Code for Sustainable Homes 5 or 6 (or Code 4 with a fabric first approach)[footnote 3] or the Passivhaus Standard[footnote 4].
This report presents further details of the study, sets out its main findings, and highlights key actions to increase demand, improve supply, and deliver low cost housing with improved energy and carbon performance.
Notes:
The report uses ‘case study’, ‘scheme’, and ‘site’ to refer to the 4 featured developments – these terms are synonymous.
The principal evaluation took place from 2017 to 2021. The report does not capture in detail more recent events such as resolution of issues identified in the case studies or the impact of the current (autumn 2022) energy prices.
2. Background and context
BEIS’ aims for this study
Building for 2050 aimed to evaluate a series of low cost, low carbon housing developments from conception to occupancy. It set out to capture lessons learned, quality, and standards achieved relating to the performance, in terms of building fabric, services, and occupancy.
More specifically, the project helps to:
- understand the barriers and opportunities of scaling up these types of homes, how to accelerate their deployment, and eliminate the requirement for future retrofit;
- understand the effect they could have on UK energy demand and carbon emissions;
- gather evidence for current and future standards (both regulatory and voluntary);
- appreciate the process end-to-end and incorporate the views of multiple stakeholders. Previous research in the sector had mostly focused on discrete elements.
This was with the overall goal of new homes being near-zero or zero carbon by 2050 – even though the start of the project preceded the Net Zero target. While this and other strategies, policies and circumstances have changed since the start of the project, the fundamental aims have remained the same.
New low carbon requirements
Building for 2050 commenced in September 2017. It was commissioned at a time when there was no national regulatory driver for low carbon homes and there was a greater focus on deregulation. The government had cancelled its policy of zero carbon homes for 2016 and the Code for Sustainable Homes[footnote 5] had been withdrawn.
The project has become increasingly relevant in recent years to support the renewed focus on the reduction of carbon emissions in new homes. There is now a requirement in the Climate Change Act for all greenhouse gas emissions in the UK to be net zero by 2050,[footnote 6] and the government’s plan for meeting this target has been set out in its Net Zero Strategy.[footnote 7] To help meet this requirement, the government has consulted on a Future Homes Standard for England, to be brought into force in 2025. In December 2021, it published the Part L 2021 standard[footnote 8] as a stepping stone to the Future Homes Standard. Wales published changes to Part L in May 2022[footnote 9] and consultations are taking place in Scotland[footnote 10] and Northern Ireland[footnote 11], all of which should substantively reduce carbon emissions from new homes. Some local authorities are setting planning requirements that go beyond Building Regulations to deliver low carbon homes faster. Hence, significant market drivers now exist for low carbon homes. The challenge is to ensure that these homes are affordable, deliver low carbon performance and are attractive to residents. This study provides key insights on how this challenge can be met.
We will increase standards for new builds in the 2020s to ensure that they are ready for Net Zero
In 2019, the government announced the introduction of a Future Homes Standard for England by 2025. The standard will ensure that new build homes are future-proofed with low carbon heating and high levels of energy efficiency. We expect that homes built to this standard will produce no operational carbon dioxide (once the supply has been decarbonised), with 75% to 80% lower emissions than those built to current Building Regulations standards.
(BEIS Heat and Buildings Strategy)
Opportunities arising from grid decarbonisation
New strategies are needed to meet low carbon standards. Many low carbon homes will use electricity for heating, given the planned decarbonisation of the grid (see Figure 1 which shows the projected carbon intensity of the grid). This is in line with the government’s Net Zero Strategy and Heat and Buildings Strategy, which suggest heat pumps will be the most appropriate low carbon heating option for new buildings[footnote 12]. While all the case studies used electricity for heating, it is important to emphasise that alternative technologies could be used.
Figure 1: Projections of power sector carbon intensity by 2050
(Based on National Grid, Future Energy Scenarios 2021, Figure SV.27. Includes negative emissions from bioenergy with carbon capture and storage. Note that the carbon intensity in 2017, when this study commenced, was around 260gCO₂e/kWh.)
Description of Figure 1:
Graph showing projections of power sector carbon intensity from 2020 to 2050. See text below for details.
Increasing focus on whole life carbon (WLC)
The study focused on designs targeting a reduction in regulated[footnote 13] operational carbon. However, there is growing interest in whole life carbon (WLC), recognising additional sources of carbon and their role in meeting the net zero target[footnote 14]. WLC emissions goes beyond operational carbon, covering emissions resulting from the construction and use of a building over its entire life, including demolition and disposal. While some of the key insights from the study extend to all carbon emissions, further activities are necessary to achieve net zero accounting for all carbon emissions. This is discussed further in Section 9.
Retrofitting existing homes
Most existing homes will need energy efficiency upgrades (in some cases significant works) and all homes will need low carbon heating in line with the Net Zero Carbon commitment. Many of the learning points identified from this research are relevant. These include delivering homes that achieve their designed energy and carbon performance, focusing on running costs as well as carbon; and implementing solutions that are attractive to residents through being easy to understand and operate.
3. Approach
Overview
The Building for 2050 research project comprises a literature and market review, 4 in-depth case studies of low carbon developments, and interviews and surveys of wider technical and consumer stakeholders. Each of the activities is detailed below.
Literature and market review
A desk-based review was undertaken at the start of the project, comprising:
- a market review of low carbon housing constructed currently;
- a literature review of previous social research and discussions with key academics around the views of the housing industry on delivery of low cost, low carbon homes and the experience of residents living in these homes;
- a literature review of the challenges faced when delivering low carbon homes and solutions to improve build quality and address the performance gap;
- an assessment of how low carbon homes fit within a changing energy system;
- The insight gained in these reviews helped frame our research and analysis with further work, undertaken towards the end of the project, to capture current policy drivers and more recent, significant industry guidance.
In-depth case studies
Four developments in England and Wales were studied in detail from project inception through to occupancy and experience in use, see Figure 2. This provided insights from those stakeholders both making key decisions and delivering the works. It also allowed an examination of how the whole process was delivered.
BEIS originally recruited 3 developments prior to the research commencing, but only one of these proceeded to construction and a further 3 developments were recruited. This proved a challenge: the development timetable needed to align with that of the research project, and some developers of suitable schemes were not willing or able to participate.
Figure 2: Location of case studies
Description of Figure 2:
Map of the UK showing the locations of case studies:
- Etopia Homes - Northamptonshire
- Marmalade Lane - Cambridge
- Tallack Road - Leyton, London
- Active Homes - Neath, South Wales
Copyright for images:
- Etopia Homes © Etopia Homes
- Marmalade Lane © David Butler
- Tallack Road © Galliard Homes
(The Active Homes photo was taken by a member of the research team)
The 4 developers had different funding models and a wide range of drivers for the schemes they developed. They also adopted different approaches to low carbon design. Combined with the variation in tenure, this provided a good range of data for the research.
Each of the case studies and their low carbon features are described later in this section (see case study schemes).
Each case study typically comprised the following activities:
- social research with the development team. Semi-structured interviews included questions around the drivers, barriers, attitudes, and challenges with the delivery of low cost, low carbon homes. It included the views of funders, developers, design teams, contractors, and site-based staff;
- social research with residents. It included both the expectations of living in low carbon homes and a comparison to the experience in practice. It included semi-structured interviews and focus groups;
- a design review of the homes and subsequent site observations during construction, and post-completion, to highlight key differences from the design and identify reasons for any significant changes;
- an assessment of the additional capital costs associated with delivering the low carbon solution;
- post-completion testing of the thermal performance of the building envelope and the performance of any mechanical ventilation systems;
- a comparison of the SAP modelling by the development team with that undertaken by the research team, accounting for any differences identified;
- monitoring of the energy and environmental performance of homes post-occupation. This included: sub-metered energy use; temperature, relative humidity and CO₂ monitoring; and residents’ use of windows. Residents also completed monthly diaries to record key activities that could impact on energy use and internal environmental conditions, such as going on holiday;
- identifying how the low carbon homes fitted within a changing energy system.
Wider stakeholder engagement
Surveys to determine the views of wider groups were also undertaken to provide a broader perspective:
- 36 semi-structured interviews were carried out with technical stakeholders to explore different perspectives on the production of low cost, low carbon housing. They were selected to have a range of experience and knowledge of housing from funding, commissioning, designing, constructing, or regulating new build projects;
- a UK online survey was carried out to capture the views and experiences of a wide range of consumers in relation to sustainable homes. A total of 2,500 consumers were included in the research, including a mix of 50% buyers and 50% renters.
In addition, other stakeholder engagement events were undertaken:
- a workshop was held that focused on the challenges and solutions to deliver low carbon, low cost housing which works for residents. Attendees included technology providers, service providers and retailers, and development solutions providers;
- emerging findings were presented and discussed at several events, including at Futurebuild 2019, 2020 and 2022, and via a Good Homes Alliance-organised event;
- a webinar was held towards the end of the study, with around 200 attendees. They comprised developers, designers, architects, consultants, government, local authorities, technology companies, service providers, electricity and gas network companies, and academia. Findings were presented and attendees surveyed around some of the emerging conclusions from the project to feed into this report.
Case study schemes
The following pages describe each case study and their key low carbon features.
Marmalade Lane, Cambridge
Provides 42 dwellings, a mix of flats and houses, with a shared ‘Common House’ facility. It deploys a fabric-first design with offsite timber panels for houses and paired ‘Tyneside’ type flats, and pre-fabricated CLT panels for the main block of flats and the Common House. Low carbon features include a triple-glazed panel system, ASHPs to supply heating and hot water, and MVHR.
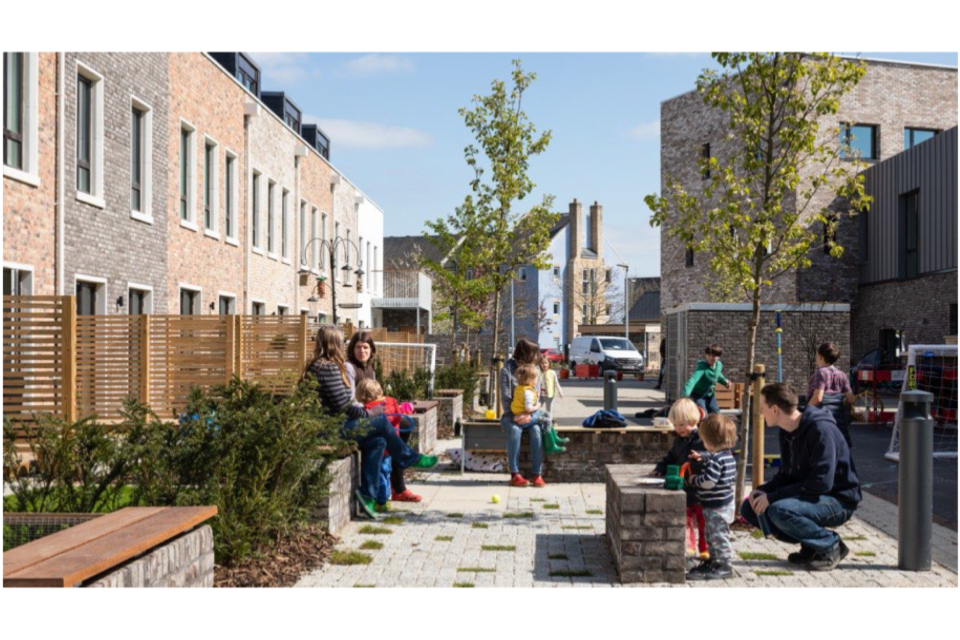
© David Butler
Team
Developer: TOWN and Trivselhus UK
Architect: Mole Architects
Consultants: Hoare Lea, Elliott Wood, Etude
Low carbon technology
- Air source heat pumps (ASHPs)
- Mechanical ventilation with heat recovery (MVHR) system
Construction method
Trivselhus Climate Shield closed timber panels for the houses and paired Tyneside flats. Prefabricated cross-laminated timber (CLT) panels for the main apartment and Common House block.
Date of completion
December 2018
Specification
-
Air source heat pump
-
Cylinder and buffer tank
-
Underfloor heating
-
Radiators in upper storeys with thermostatic radiator valves (TRVs)
-
MVHR unit
-
Timber frame
-
Triple glazing
-
Electric cooking
-
South facing roof to accommodate future installation of solar photovoltaic (PV) panels.
Active Homes, Neath, South Wales
Provides 16 homes, a mix houses and flats, for social rent. Grant-funded low carbon features include: off-site manufactured timber frames and double glazing; PV panels with battery storage, and MVHR. The homes also include hot water from individual ASHPs preheated by Transpired Solar Collectors (TSCs) integrated into the building envelope.
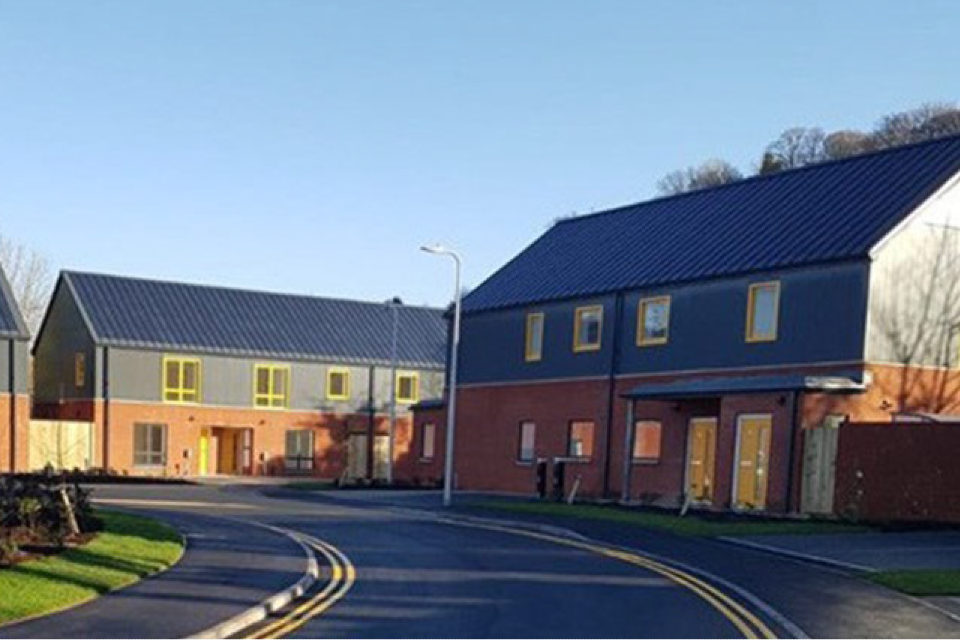
Team
Developer: Pobl Group
Architect: Pentan Architects
Consultants: Asbri Planning, Auxilium, ER Brown
Innovation Partner: SPECIFIC
Contractors: T Richard Jones Ltd. / MMC supplier: Sevenoaks Modular
Low carbon technology
- Air source heat pumps to generate hot water with pre-heat from transpired solar collectors
- Mechanical ventilation with heat recovery system
- Integrated roof mounted photovoltaic panels
- Individual battery storage
- A+++ rated appliances and LED lights
- Electric vehicle charging points
Construction method
Structurally insulated panels (Timber SIPs) with independent facing brickwork, polyester powder coated metal cladding, and standing seam roof.
Date of completion
November 2019/November 2020[footnote 15]
www.poblgroup.co.uk/our-developments/active-homes
Specification
-
MVHR unit and in-line on-peak electric heater
-
Transpired Solar Collector (TSC) - Perforated steel cladding acts as a TSC, pre-heating the air inflow to the domestic hot water system
-
Roof integrated solar PV
-
ASHP water heater with integrated cylinder
-
Electric vehicle charge point
-
Battery storage - Householder’s use of solar generated electricity is maximised by battery storage
-
House switchboard
-
Electric cooking
-
Timber frame
There was a later change to the form of space heating.
Etopia Homes, Corby, Northamptonshire
Provides 47 homes, a mix of houses and flats, for private sale. The scheme has a wide range of low carbon technologies including a structurally insulated panel construction, triple glazing, a Ground Source Heat Pump (GSHP) for heating and hot water, and an Earth Energy Bank providing inter-seasonal storage (and a heat source for the pump). The design also includes a PV-Thermal hybrid solar array, MVHR, and a Smart Home Energy Management (SHEM) system to co-ordinate the technologies. ASHPs were installed on the last 21 houses; only the first 10 had a GSHP. Infra-Red electric panel heaters for space heating, and ASHPs for DHW, were installed in 16 apartments.
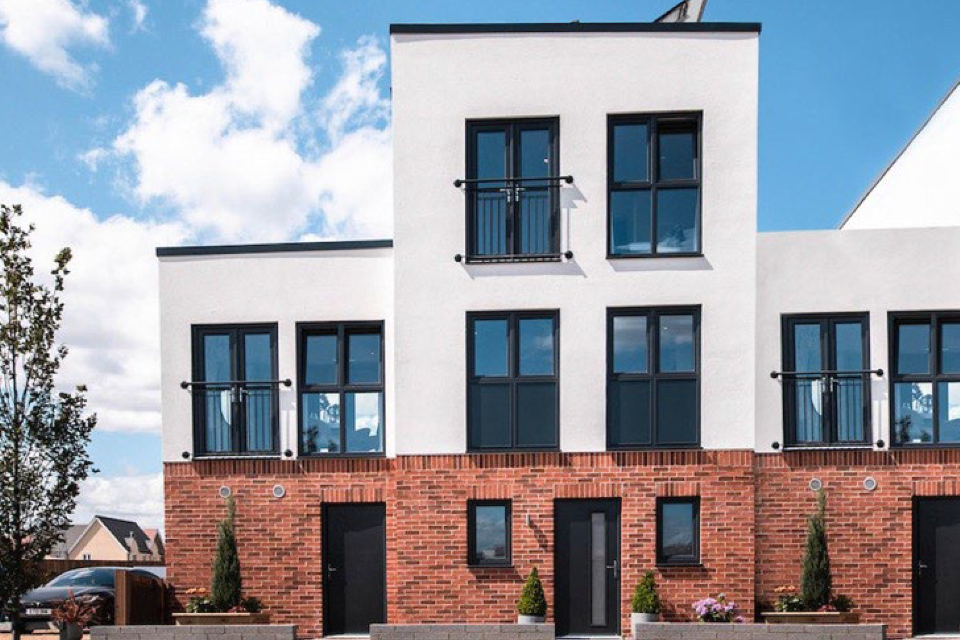
© Etopia Homes
Team
Developer: Glendale Ecohomes (RIBA stage 1-2), then Project Etopia (RIBA stages 3-7)
Architect: Staniforth Architects
Consultants: Electric Corby, Carbon Free Group, Smart Power Systems, Caplin Solar
Low carbon technology
- Earth Energy Bank (EEB) storage and Ground source heat pump (GSHP
- Photovoltaic-Thermal hybrid solar array (PV-T)
- PV array
- Mechanical ventilation with heat recovery system
- Smart Home Energy Management (SHEM) system
Construction method
4Wall structurally insulated panel (Steel SIP) construction with render and brick slips externally.
Date of completion
Phased completion. First homes occupied January 2020.
Specification
-
Roof integrated Solar PV & Thermal (PVT)
-
MVHR unit
-
Radiators in upper storeys
-
Wastewater heat recovery
-
Smart home energy management system
-
GSHP water heater with integrated cylinder
-
Battery storage - Householder’s use of solar generated electricity is maximised by battery storage and exported to the grid
-
House switchboard
-
Electric cooking
-
Underfloor heating on ground floor
-
Earth Energy Bank - Solar energy heats a liquid pumped into underground pipes. This heat is pumped back into the home when needed in winter
-
Modular SIP construction and triple glazing
Later homes adopted a different specification.
Tallack Road, Waltham Forest, London
A private development of innovative low carbon housing designed to comply with the London Plan’s carbon emission targets. It provides a mix of 80% private and 20% affordable homes in 48 flats and 2 houses. The low carbon features include a communal ambient loop heat network that provides heating and hot water using ASHPs, in-dwelling water-to-water heat pumps, and centrally located PVs that feed the landlord’s supply.
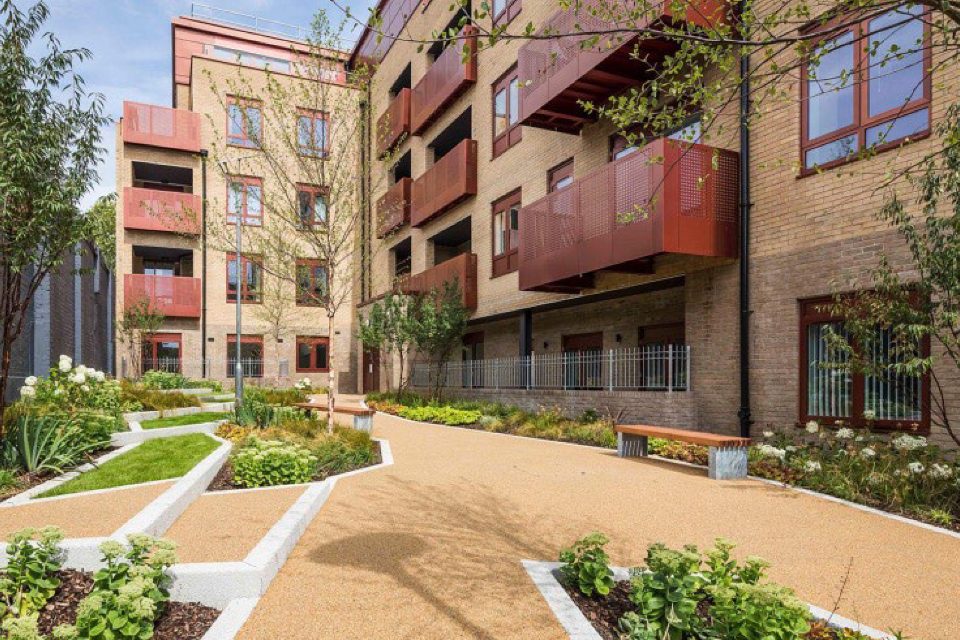
© Galliard Homes
Team
Developer: Galliard Homes
Architect: Osel Architects
Consultants: EDC London
Low carbon technology
- Roof-level photovoltaic panels (PV)
- Communal ambient loop ASHP heating system with in-dwelling water source heat pumps
Construction method
Traditional brick construction.
Date of completion
March 2020 www.galliardhomes.com/church-road
System
-
External communal ASHP unit
-
Thermal stores in plant room
-
Ambient communal heating loop
-
Apartment water source heat pump
-
Apartment hot water cylinder
-
Radiator distribution system
-
Individual room thermostats
-
MEV inflow via trickle vents
-
MEV outflow unit
-
Solar PV panels
-
Electric cooking
4. Headline findings from each case study
The key findings from each case study are presented in the following pages.
Marmalade Lane
Overview
(Figure 3 shows the key design issues encountered at each stage of the development)
- A joint venture between a private developer (TOWN) and an off-site timber panel manufacturer (Trivselhus, who also funded the project) for private sale to members of a co-housing group (Cambridge Cohousing Ltd);
- 42 homes, a mix of flats and houses, with a ‘Common House’ providing shared facilities;
- Adopted a fabric-first approach with offsite manufactured closed timber panels (houses) and pre-fabricated cross laminated timber (CLT) panels for the apartments;
- Low carbon features: panel system with triple glazing, ASHPs to supply heating and hot water (individual ASHPs in houses and communal ASHPs for apartments and ‘Common House’), MVHR.
Main drivers and barriers for the supply of low carbon homes
Main drivers:
- Decision by landowner (Cambridge City Council) for a community-led approach to this site
- K1 Cohousing was set-up in response. They were key decision makers in forming the brief. Key principles were to deliver high environmental standards, including Passivhaus performance
- A requirement from the future residents to avoid any use of gas on site
Main barriers:
The main barriers were the extra capital cost and lack of a Passivhaus supply chain.
- No affordable tenders returned for intended Passivhaus standard. K1 Co-housing accepted 70% of Passivhaus space heating standard as reduction against applicable Part L
- PV panels were dropped from the design to retain a low carbon design at viable prices
- At construction stage, the 70% Passivhaus standard was replaced with a 30% CO₂ reduction on Part L 2013. A performance standard (kWh/m²/yr) was not retained
Delivery
Performance:
- The measured space heating fuel use for both houses and flats is on average around 3 times higher than the developer SAP estimate;
- These results are partly reflective of the actual installed performance of the building fabric, for example, actual airtightness 3 times poorer than the value in SAP and issues with thermal bridging. The results showed good thermal performance of the pre-fabricated panels;
- The results also suggest the communal heat pumps serving the main CLT apartment block are significantly less efficient than design expectations, caused by missing distribution system components and sub-optimal commissioning.
- Energy use for Domestic Hot Water (DHW) was lower than predicted: this may reflect most homes having lower occupancy than the SAP assumption,
- A range of issues occurred relating to ventilation (equipment and design strategies), causing overventilation and poor energy performance, but good air quality;
- Sufficient electrical capacity present – electrical infrastructure upgrade not required;
- The actual carbon emissions for houses are estimated to be typically around 15% better than the Part L 2013 target. In comparison, carbon emissions for CLT flats are around 10% higher than the Part L 2013 target.
Cost:
- Delivered at market prices and generating a profit for the developer, although not at normal market level;
- The initial brief from the co-housing client group was amended with the group’s agreement to balance capital cost and affordability;
- The timber panel system was provided to the contractor under a nominated sub-contract agreement free of charge, as the manufacturer was a Joint Venture partner;
- The additional cost for design, supply and install of low carbon technologies was 2.5% of construction cost.
Residents’ experience
Main drivers:
- Attracted by the multi-cultural and multi-generational community;
- Sustainable design, energy efficiency and low energy bills of the properties;
- The location of the properties in Cambridge.
Main barriers:
- Delays in seeking a developer and then in determining planning, accompanied by cost increases reflecting price and costly inflation in the local housing market;
- Homes moved away from Passivhaus at concept stage to targeting a space heating demand of 35kWh/m²/yr. The performance standard was downgraded at the construction stage, with capital cost the focus;
- Concern about the effectiveness of the low carbon technologies selected and how easy they would be to use and maintain;
- The location (while attracted to Cambridge, some residents had concerns about the specific location).
Main benefits of living in their home:
- Residents are generally pleased with their homes and regard them as high quality;
- Warm and comfortable (not draughty);
- Good performance of the ventilation system, good air quality with absence of condensation and mould.
Main challenges of living in their home:
- Lack of training on how the systems function and how to best operate them. This is despite support of other residents;
- Some disappointment that homes were Energy Performance Certificate (EPC) band B rated, not band A;
- Many felt energy bills were higher than expected. Residents were unclear if this was due to the performance of the homes or if they were being used sub-optimally;
- Overheating issues which required active management by residents.
Figure 3: Key issues encountered at each stage of development – Marmalade Lane
Pre-design factors
Drivers:
Land allocated for standard open market housing.
Local planning targets in place.
- 10% reduction in carbon emissions beyond Part L
- Renewable energy to meet 10% of predicted energy requirements
Co-housing group received assitance to develop the brief at no cost to them.
Site layout and orientation optimised for passive design.
Co-housing group specified low carbon homes - certified Passivhaus1 performance, CSH2 level 4, no gas at the development, PV and solar thermal panels3.
Design
Developer competition held.
Low carbon adviser role was not funded once developer was in place.
Design progressed as per concept stage, but Passivhaus target replaced with ‘70% Passivhaus’ which aligned with the Trivselhus Climate Shield system4.
Additional cost for Passivhaus-certified homes estimated to be £10k per dwelling.
Fabric-first approach adopted, but building services design changed to reflect higher space heating demand.
CSH level 4 energy target5 retained but full level 4 was dropped6.
Initial design not intended for timber panel approach. Apartment building and ‘Common House’ later changed to a CLT structure with similar insulation standard.
Agreed specification: 70% Passivhaus, no gas, heat pumps, MVHR7, future-proofed for PV.
PV panels axed at detailed design to reduce Capex.
ASHPs provide space heating8 and hot water.
Solar thermal not required for DHW/renewable energy planning target. Houses had 300 litre DHW cylinders.
Modelling did not identify a significant overheating risk.
Procurement
Design and Build contractor selected for its experience with the construction method - not experienced in residential sector or with low carbon technologies.
Performance target revised to 30% better than Part L (no Passivhaus requirement and no kWh/m2/yr target).
Trivselhus system retained (provided free of charge to the contractor).
Benefits of enhanced building fabric not reflected in Mechanical & Electrical (M&E) services design. Houses had underfloor heating (ground floor) and radiators in hall.
Tender specification overly complex and prescriptive. ASHPs were oversized.
Limited handover requirements included within tender.
Construction
Insufficient design co-ordination prior to start on site (M&E design lagged architectural design).
Site logistics and layout not planned for delivery of pre-fabricated elements.
Constructed to programme with minor delays in part due to local labour shortages - brick external leaf.
Flat roof and sloped roof had less insulation than specified.
Building airtightness did not meet design intent, and thermal bridging was worse than design assumptions, but better than Interface Unit and MVHR took longer to industry standard.
Together the ASHP and MVHR installation took considerably longer than a gas boiler and extract fans (2 weeks vs a few days).
Issues with installation of MHVR - size, weight, selected location at top of stairs.
Ductwork complicated and lengthy, reducing efficiency and in some cases clashing with roof insulation.
Handover, commissioning, snagging
Some incorrect commissioning of equipment due to error, complexity, and lack of understanding.
Some snagging issues arising from low carbon features - for example, poor airtightness of triple glazed patio doors (not incorportaed within the panel).
High comissioned ventilation rates, rather than optimal - this is an industry issue.
Minimal hadover provided. Services were more complex than in standard or Passivhaus-certified homes.
Residents were disappointed with extent of handover but found co-housing ethos helpful to resolve issues.
Significant issues with communal ASHPs - commissioning, tariff. Some relocated from roof to avoid noise and vibration issues.
Occupation
Residents were pleased with their new homes and liked being part of a co-housing community.
- The homes wee warm and comfortable
- The homes had low energy use
- Residents liked design and layout
- Residents felt they were able to live more sustainably
Residents faced a few challenges - higher than expected bills, systems challenging to operate optimally, some interior space constraints, some found air too dry.
Equipment had independent controls - space heating (2 forms in houses) and ventilation systems.
Higher bills caused by:
- Dwellings not quite performing as intended (performance gap for fabric and services) and challenging to control
- Particular performance issues with the communal ASHP system
- Electricity unit cost being much higher than for gas, limited tariffs, no on-site electricity generation (PV panels dropped from specification)
Some residents experienced overheating. Some physical solutions deployed (for example, solar control film) and some behaviour changes.
Some residents retrofitted PV panels.
Many of the initial snagging issues were resolved by year 2 of occupancy and residents also better understood how to optimise their systems.
1. Certified Passivhaus requires space heating of 15kWh/m2/yr or less.
2. CSH = Code for Sustainable Homes
3. The form of heating system was not specified.
4. Trivselhus were a joint venture development partner. Its Climate Shield product comprised a closed panel timber frame with triple-glazed windows. It delivered similar to Passivhaus fabric standards but did not include Passivhaus-certified windows. Performance standard agreed was 35kWh/m2/year on average.
5. CSH level 4 energy target required a 19% reduction on Part L compliance.
6. The co-housing group did not want to accommodate some of the core Lifetime Homes requirements because of the design constraints and cost implications.
7. There was a planning condition related to the MHVR, due to concerns about the nearby major road.
8. Space heating loads were increased in line with the higher space heating target.
Active Homes
Overview
(Figure 4 shows the key design issues encountered at each stage of the development)
- Developed by Pobl, a social landlord focused on innovation, fuel poverty and the reduction of dwelling carbon footprint;
- 16 dwellings (flats and houses) for social rent with timber-framed modular SIP system;
- Low carbon features: off-site manufactured timber frame and double glazing, electric panel heating, hot water from individual ASHP preheated by TSCs integrated into the building envelope, PV panels with battery storage, MVHR;
- Grant funding covered additional costs of low carbon features.
Main drivers and barriers for the supply of low carbon homes
Main drivers:
- Shared vision of Local Authority (landowner), developer, and innovation partner (design concept) for homes with low energy use and bills, and low environmental impact;
- Grant funding to allow inclusion of a range of innovative technologies.
Main barriers:
- Capital cost for innovation technologies;
- Given the small infill nature of the site and existing significant trees and planning constraints around orientation and active frontages, the solar access was limited for some properties, thereby reducing the energy generation.
Delivery
Performance:
- The measured space heating fuel use for houses is on average around 3 times higher than the developer SAP estimate. In comparison, for flats the measured space heating fuel use is around 2 times higher than the developer SAP estimate;
- These results partly reflect the expected thermal bypass issues between Modern Methods of Construction (MMC) walls and conventional roofs. The results showed good thermal performance of the pre-fabricated panels;
- In addition, MVHR located in cold lofts increased heat loss and resulted in cooler residential supply air;
- The space heating system had insufficient capacity at completion and resolving issues was costly and took significant time and cost;
- Energy use for domestic hot water (DHW) was lower than predicted; this may reflect adapting to lower-than-expected availability of hot water and the contribution of renewable heat from TSCs (not included in SAP predictions);
- The actual carbon emissions for houses and flats are estimated to be 60% and 85% below the Part L 2013 target, respectively[footnote 16].
Cost:
- Additional cost for design, supply and install of low carbon technologies was 18% of construction cost;
- Supported by grant funding from the Welsh Government Innovative Housing Programme (IHP).
Residents’ experience
Main drivers:
- The location of the properties;
- The interior and exterior design and layout of properties; for example, for residents with health issues, a home with good accessibility was a key priority;
- In general, the sustainable nature of homes was not a primary driver.
Main barriers:
- Concern of having novel technologies and preference for a more traditional home with more familiar systems;
- Not having gas in the homes as they were used to gas central heating and cooking with gas;
- Delays to occupancy due to the issues with the low carbon technology.
Main benefits of living in their home:
- The design, layout, and overall build quality of the homes are considered to be key benefits;
- Air quality has been good, with very few issues with damp or mould;
- Most are happy with their electric cooking facilities and feel safer in their homes without gas, but some feel cooking and heating with gas is quicker and more effective.
Main challenges of living in their home:
- Concerns of insufficient handover (during Covid). Understanding the heating and ventilation system was an ongoing issue;
- Hoped-for lower energy bills. Some had significant concerns about how high they were (key cause is direct electric heating);
- Some had insufficient hot water. A key reason is the heat pump recharges the cylinder more slowly than a gas boiler would;
- Cool draughts from ventilation system.
Figure 4: Key issues encountered at each stage of development – Active Homes
Pre-design factors
Pobl’s desire to deliver low carbon homes, tackle fuel poverty and deliver resident satisfaction.
Local innovation partner (SPECIFIC) had ‘Buildings as Power Stations’ concept (conserve, generate, store, release)1.
Shared local vision (local authority, Pobl and SPECIFIC) to deliver low carbon homes and support development of a local supply chain.
Desire to develop a pilot scheme for wider application via Swansea City Deal funding application.
Grant funding from Welsh Government (WG) provided gap funding for additional design and capital costs.
Lack of development experience on behalf of the innovation partner.
Design
Concept design: a high-performance fabric, renewable technology and energy storage - focus on innovation.
Infill site constraints and planning authority’s design principles limited on-site energy generation.
Change from Buildings as Power Stations to ‘Active Homes’ concept.
Design: modular timber fabric, MVHR, warm air heating (heater battery), TSC pre-heat to ASHP for DHW, roof integrated PV, communal electric battery.
Warm air heating did not drive required building fabric performance standard.
Communal battery replaced by individual batteries.
M&E design lagged architectural design.
MVHR and heater battery located in loft but design change from warm to cold roof and implications for heating provision not fully understood.
Challenges:
- Extra time and fees required for design
- Extra capital cost of innovative technology
- Extent of innovative technologies and complexity of integration between technical approaches
- Time constraint imposed by grant funding for entering Design and Build contract
- Low carbon adviser and M&E consultant lacked housebuilding experience
- Low carbon supply chain offered limited choice of some M&E products
- Insufficient design co-ordination prior to start on site
Procurement
Tender specification broadly as design, emphasis on local suppliers.
Warm air heating system approach not deliverable through domestic-sector products (MVHR unit and heater battery not compatible).
Limited choice of ASHPs to supply DHW only, rather than space heating and DHW.
Limited low carbon supply chain influenced sub-contractor selection.
Contractor inexperienced with low carbon technologies.
M&E consultants not retained to oversee site works due to budget constraints. Importance of role not fully understood by client.
Construction
Sub-contractors inexperienced with low carbon technologies.
Issues resolved on site without full understanding of performance impacts.
Site co-ordination challenges, some thermal bridging observed.
Ducting design clashed with insulation; a greater issue due to modular timber frame system.
PV installation issues.
MVHR, heater battery, TSC ducting and cowl challenging to install.
Dual-phase electrical supply required in dwellings which added complexity and cost, and also required specialist sub-contractors2.
Dual-phase electricity enabled electric vehicle (EV) charging points, a late addition.
Handover delayed by significant drainage issue.
Retrospective planning application needed for roof cowl.
Pobl had concerns regarding underheating and likely bills.
Handover, commissioning, snagging
Building fabric generally performed well and airtightness close to target, but insufficient on-site QA (some thermal bridges and missing loft insulation).
Poor commissioning of MVHR (industry-wide issue) influenced by the heating strategy.
Underheating issues identified prior to occupation. Changes made to warm air heating system but underheating persisted.
New installation required, comprising electric panel heaters in habitable rooms with radio frequency controllers. Air supply changed to delivery ventilation only (not space heating as well).
Building Integrated PV made metal roof live for one block. Lightning protection added and adjustments made to PV.
Approx. 12 months delay to occupancy.
A significant thermal bypass deemed highly likely3, so heated air passes directly to cold loft space.
Planned handover not delivered, limited by COVID-19.
Occupation
Positive feedback on design, layout and build quality.
Most residents had higher than expected energy bills. Some had deep concerns about affordability.
Space heating was a challenge to operate, views on thermal comfort were mixed.
Some reported that the DHW supply was insufficient and/or too slow to recharge.
Most experienced issues with ventilation system (units changed to low setting or off).
Wintertime energy use significantly exceeded on-site generation, leaving no excess electricity to store.
Homes designed for time-of-use and export tariffs to reduce bills, but this required smart meters. No smart meters were available for dual-phase electrical supply. Therefore, bills were higher than planned.
Energy consumption likely to vary significantly across seasons due to on-peak electric space heating and lower withertime generation of electricty by PV.
Pobl continued to investigate performance issues and was partly paying residents’ electricity bills.
1. The Buildings as Power Stations concept and potential benefits are summarised in ‘One Million Homes Constructed as ‘Buildings as Power Stations’’ – Report of Indicative Benefits, SPECIFIC, 2017.
2. Typically, single electrical phase power is sufficient for the electrical loads of a house. Dual or 3-phase allows some circuits in the house to operate on a different phase of the alternating current to increase the total supply capacity and might be required, for example in all-electric homes, It requires upgraded infrastructure to be installed. In this case, the all-electric homes with a heat pump, electric cooking, solar PV, and a battery were deemed to exceed the limit for single phase supply. A 3-phase supply was installed to the site and homes, but only 2 phases were needed and used, via 2 x distribution boards within the homes. All equipment is single phase.
3. By the research team.
Etopia Homes
Overview
(Figure 5 shows the key design issues encountered at each stage of the development)
- 47 homes (flats and houses) for private sale;
- Initial development team was a consortium, Glendale Ecohomes;
- Designed as a low carbon scheme within a much larger development, responding to a local vision. The low carbon design was driven by innovation and a wish to create a new low carbon housing product;
- Low carbon features of the first 10 homes: structurally insulated panel construction; triple glazing; GSHP to provide space heating and hot water; an Earth Energy Bank (EEB) under each home to provide inter-seasonal storage and be the heat source for the GSHP; Hybrid solar array to charge the EEB and generate electricity; PV linked to battery storage; MVHR; SHEM system to co-ordinate the innovative technologies;
- £21k grant funding obtained per dwelling towards the additional £30k equipment costs;
- Once planning permission was obtained, the consortium’s inability to attract development funding resulted in a change of developer, with Project Etopia (PE) taking over and delivering RIBA stages 3 to 7;
- Later dwellings within the scheme had an amended low carbon design, in part driven by the grant funding no longer being available for these units.
Main drivers and barriers for the supply of low carbon homes
Main drivers:
- Initially driven by a community interest company, set up with the support of the local council to promote local low carbon living;
- Initial concept of ‘energy positive’ PV-Thermal (PV-T) homes with zero energy bills;
- Innovation funding attracted to test novel system to deliver low carbon performance.
Main barriers:
- Obtaining investment for developing low carbon homes.
a. New developer purchased the site from the initial developer, who could not attract the funds to build the scheme due to the combination of innovative technologies and potential lower returns, and their lack of volume house building experience;
b. The new developer found it challenging to attract investment as a new company and new market entrant. They had to deliver and sell initial homes quickly to fund subsequent ones. - Detailed design was being developed during construction and the developer adopted a ‘learning by doing’ approach;
- The electrical network needed to be upgraded for the all-electric homes;
- A simpler, lower-cost, low carbon specification was adopted for the final 21 homes[footnote 17] that were ineligible for grant funding due to a timing limitation. These homes had ASHPs (houses) or radiant panels for space heating and ASHPs for hot water (flats). They are reported by the developer as performing much better than the first 10 homes with GSHPs.
Delivery
Performance (based on those dwellings with GSHPs):
- Relatively late introduction of a modular build system created design and construction challenges. The product offered a restricted range of panel types at the time;[footnote 18]
- In general, post-completion testing suggested the building fabric U-values performed similarly to design specifications, but there was poor integration of wall panels with other built elements, leading to poorer airtightness than predicted;
- Insufficient design co-ordination impacted on performance. A change to the floor construction impacted the EEB performance;
- The in-use energy data intended to be supplied by the developer was not available.
- The predicted annual space heating fuel use for a house, based on the research team’s as-built SAP, was around 2.5 times higher than the developer SAP estimate. The research team predicted the domestic hot water use to be similar to the developer SAP estimate;
- Significant underperformance of low carbon technologies operating within a novel and complex system;
- Bills impacted by reduced efficiency of the space heating system and residents not having access to time-of-use and export tariffs (a certification and metering issue). Third party management of the energy in the homes, via the SHEM, as initially planned, should assist in reducing bills;
- The actual carbon emissions are estimated to be typically around 85% below the Part L 2013 target.
Cost:
- Additional cost for design, supply and install of low carbon technologies was 9% of construction cost;
- Some of the low carbon technology, the EEB, was paid for by funding from the European Horizon 2020 Programme;
- Homes were sold at the market rate, accounting for the fact dwellings were around 25% larger (m²) than would be typical for the number of bedrooms provided.
Residents’ experience
Main drivers:
- Location of the properties;
- Attracted to the interior and exterior design and layout of the properties;
- Sustainable and energy efficient nature of the properties and the prospect of having low or zero energy bills.
Main barriers:
- Concern of actual performance and reliability of homes and technologies used. Not being as energy efficient as predicted;
- Expectation of overheating during warmer periods due to the high standard of insulation and the number of windows.
Main benefits of living in their home:
- The design, layout, and aesthetics of the homes were considered to be the main benefits, especially the natural light entering the homes;
- The homes retained heat well and maintain a steady temperature during colder periods of the year.
Main challenges of living in their home:
- The energy usage and bills being significantly higher than expected;
- Residents felt they were highlighting performance issues and that these were due the project team’s lack of experience and understanding of the technologies and their integration;
- Some felt they received insufficient handover (during the COVID-19 pandemic);
- Understanding the heating and ventilation system was an ongoing issue. Belief the system was not working properly. Some missed traditional gas heating;
- Many had issues with overheating;
- All have experienced issues with hot water running out or not reaching the desired temperature;
- Mixed views on the indoor air quality and ventilation.
Figure 5: Key issues encountered at each stage of development – Etopia Homes
Pre-design factors
Local authority had a desire to promote low carbon regeneration of local economy.
Community Interest Company developed the brief of ‘energy positive homes with ‘zero energy bills.’’
Consortium formed to develop the scheme which included a low carbon advisor.
Low carbon advisor developed innovative low carbon concept to meet the brief.
Grant awarded for design and capital costs of some aspects - to meet the brief.
Grant awarded for design and capital costs of some aspects - PV-T, GSHP and EEB.
Intention was the low carbon consultatnt would be retrained to manage energy use across the scheme.
Design
Concept design included a high-performance timber frame, renewable energy technologies and energy storage.
Design was developed: modular 4Wall system, MVHR, PV-T, GSHP, EEB, battery, wastewater heat recivery, and SHEM.
Scheme submitted to planning was changed to be a steel panel system - the 4Wall system.
Integration between technologies was complex.
Some design changes made - ground floor design (inadvertently impacted on EEB performance), pitched roof replaced by flat roof on some homes to increase PV output.
Extra time and fees required for design.
Insufficient design co-ordination prior to the start on site.
Lack of secure ventilation to ground floor bedrooms with patio doors fitted.
Procurement
Tender specification broadly as planning proposals.
Limited low carbon supply chain influenced sub-contractor selection.
Receiving tenders from suppliers and contractors was difficult as Project Etopia (PE) did not have a track record and there were other simpler projects in the wider development and beyond.
The more experienced sub-contractors were replaced to reduce costs.
Low carbon advisor had limited role to oversee early site works. Role ceased as importance not fully understood by PE, and there was a focus on cost reduction.
Programme for constructing later units extended beyond grant funding period, so specification updated to reduce costs.
Construction
Construction commenced before detailed design was completed.
Lack of design coordination and integration between services and building fabric.
‘Learning by doing’ approach adopted, with design changes made to later phases.
Sub-contractors had some experience with low carbon technologies but not integrating them as a combined system. Lack of M&E quality on-site monitoring.
Lack of understanding of airtight homes. MVHR ductwork challenging to install and unit located in a cold garage.
Issues resolved on site without full understanding of performance impacts.
The overall system required much more space and so was located in the garage or large plant room.
Service penetrations through the SIPs1 reduced the airtightness.
Handover, commissioning, snagging
Building fabric perfomed well in terms of U-values. Airtightness was poorer than target but still better than industry standard.
The GSHP supplied space-heating and hot water. This was pre-heated by the EEB to increase efficiency, with the EEB itself supplied by PV-Thermal panels on roofs. Under-performance of this system was a significant problem.
MVHR generally performed well and was correctly commissioned for dwelling size. It needed recommissioning for smaller households.
Lack of understanding of time-of-use and export tariffs (metering, certification and provider) and required elements.
Battery installed without MCS2 certification, commissioned to import lower cost electricity from the grid overnight but this was not available.
Smart Home Energy Management controls system did not operate as intended, resulting in higher energy bills.
Monitoring of system performance did not take place as planned.
Occupation
Residents gave positive feedback on design and layout, and on winter thermal comfort.
All reported energy bills were higher than expected. Likely causes are reduced efficiency of space heating system, no access to appropriate tariffs, and no third-party energy management via SHEM.
Significant issues were experienced with the operation of the GSHP/EEB/PV-T/battery.
Residents reported being charged for electricity export rather than receiving an income, in error.
SHEM system did not work as intended.
Some overheating reported and a lack of secure ground floor openable windows (patio doors fitted to some bedrooms).
Most residents reported insufficient DHW and/or slow to recharge. The likely cause is the small size of the DHW cylinder.
Mixed views on ventilation system performance, some reported air as too dry.
SMETS2 meter retrofitted by new supplier to facilitate access to additional tariffs. Export meter and battery MCS certification provided.
Marketing changed to stress low carbon rather than low bills.
1. Structural Insulated Panels, which have a foam insulation core sandwiched between structural facings.
2. Microgeneration Certification Scheme.
Tallack Road
Overview
- Innovation-focused private developer Galliard Homes adopted low carbon heating for this scheme to comply with the London Plan carbon emission requirements;
- The scheme is a mix of 80% private and 20% affordable homes, 48 flats and 2 houses;
- Low carbon features - an innovative communal ambient loop heat network (Glen Dimplex Zeroth system) provides the space heating and hot water using ASHPs and in-dwelling water-to-water heat pumps; central PV to feed landlord supply.
Main drivers and barriers for the supply of low carbon homes
Main drivers:
- London Plan[footnote 19] Planning target of a 35% reduction in carbon emissions from Building Regulations Part L 2013 and 20% on-site renewable energy;
- Scheme with planning permission needed to be amended; no wish to use individual gas boilers;
- In-house awareness of innovative low carbon products and desire to pilot system;
- Selection of innovative ambient loop ASHP system avoided an electrical substation upgrade and delivered other benefits compared to a low temperature hot water (LTHW) ASHP communal system. Individual heat meters not required.
Main barriers:
- Concrete, steel, block hybrid construction has thermal bridging and airtightness challenges;
- Extended procurement process. The developer wanted a fixed price from sub-contractors, and given the novelty of the heating system, the system design had to be agreed with the manufacturer prior to contracting sub-contractors;
- Installation instructions for the heating system had not been tested on site so many technical queries needed to be resolved with the manufacturer.
Delivery
(Figure 6 shows the key design issues encountered at each stage of the development)
Performance:
- The measured space heating fuel use is on average around 4 times higher than the developer SAP estimate;
- The likely causes of the higher-than-predicted space heating consumption include additional fabric heat losses and inefficiencies in the ASHP installation, ongoing commissioning of central plant, and residents being unaware of how best to control their systems;
- Energy use for DHW was lower than predicted. This may reflect adapting to lower-than-expected availability of hot water;
- Developer will apply learning at future schemes with the same ASHP system – sub-metering, installation guidance, improved guidance to residents;
- The actual carbon emissions estimated to be typically 35% below the Part L 2013 target;
Cost:
- Homes sold at market rate;
- Additional cost for design, supply and install of low carbon technologies was 1.2% of construction cost;
- Innovative heating system thought to be cost neutral compared with conventional gas communal heating given the project’s scale;
- Energy bills could be lower with an enhanced fabric specification, but this would move away from a capital cost neutral approach.
Residents’ experience
Main drivers:
- The location of the properties;
- The value for money and affordability of the properties;
- Residents were generally unaware of low carbon features prior to purchase; some said it would have been a further driver had they been aware of them.
Main barriers:
- Some felt the service charge was quite high and were concerned it might increase over time;
- Lack of designated parking spaces for residents;
- Performance and reliability of the new technology;
- Learning about the new technology and how to use it;
- Not being able to visit their flat or a show home before moving in.[footnote 20]
Main benefits of living in their home:
- The design and layout of the homes;
- An expectation of lower energy bills (albeit uncertain whether energy costs were also included in service charge);[footnote 21]
- Most felt homes retain heat well and are comfortable during colder periods;
- Generally, air quality and ventilation has been good, with no damp or mould;
- All interviewed residents reported being happy with their homes.
Main challenges of living in their home:
- Learning about new systems and how to use them to optimise performance, reflecting the guidance, lack of knowledge of sales team members, and the installed controls (space heating and ventilation);
- Around half were happy with the handover. Others felt it was insufficient, especially regarding the heating system, preferring more face-to-face guidance;[footnote 22]
- Some had significant overheating issues during warmer weather;
- Some had concerns that service charges may rise because of the innovative technologies - costs they would not have control over;
- A few reported issues with hot water running out. The developer advised this was due to the domestic hot water cylinders being relatively small, although in line with the design guidance;
- Some noted the lack of heat zoning in the flats.[footnote 23]
Figure 6: Key issues encountered at each stage of development – Tallack Road
Pre-design factors
Local and regional planning policies required 35% reduction in CO2 beyond Part L 2013 and 20% on-site renewable energy generation.
Change of space heating system, post-planning.
Insufficient electricity capacity to use traditional communal ASHPs and cost of new substation would impact viability.
In-house M&E team had good knowledge of innovative products.
Goods relationship between Galliard Homes (GH) and Glen Dimplex (GD).
Design
Initial specification to meet planning targets:
- Individual gas boilers, MVHR, PV panels, improved building fabric performance
Change to specification prompted by:
- Wish to replace individual gas boilers
- Desire to avoid a new planning application
- Constraints on electrical substation capacity
- Insufficient plant space for some communal heating systems
- Desire to test innovative ambient loop ASHP system
Changed to:
- Glen Dimplex Zeroth system with central ASHPs supplying an ambient loop1 and in-dwelling water-to-water heat pumps (WWHPs)
- Mechanical Extract Ventilation (MEV) and trickle vents, smaller PV array, reduced performance target for the building fabric.
Extra capital cost of innovative technology offset against other specification changes and through avoiding a larger plant room (which would require loss of a dwelling) or substation upgrade, while still meeting the planning targets.
Reduced risk of overheating in corridors due to lower temperature of distribution system compared to typical communal heating system.
Planners accepted the change after initial concerns about visibility of the roof-top plant. ASHP rooftop location was some distance from risers.
Change to hybrid structure increased thermal bridging. Insufficient focus on the thermal performance continuity of thermal line2 and detailing.
Insufficient design coordination where specification changes were made, including building fabric and trickle vents.
Close cooperation between GD and GH at design stage and beyond. Design included in-use performance monitoring by GD.
Procurement
Low carbon expert and in-house MEP team at GD had direct participation in tender process.
Developer was able to use existing supply chain, albeit some aspects of the design were new.
GH adopted a supportive approach with its sub-contractors, noting innovative M&E design likely to cause challenges.
Hybrid construction approach increased capital costs.
Architect not retained for oversight of RIBA Stage 5 on-site.
Construction
As the architect was not retained, consequences of VE design changes and coordination issues had to be resolved on-site.
ASHP system was new, resulting in a lack of product information and system details.
External wall insulation not installed to manufacturer’s guidance.
Airtightness delivers target design performance which is industry standard.
Insufficient on-site understanding and QA of need to maintain thermal line and airtightness. Corridor heating not installed and insufficient insulation (walls between the corridor and homes or in party walls).
Unaccounted thermal bridging observed.
Handover, commissioning, snagging
GD and M&E sub-contractors worked closely with GH on commissioning of the ASHP system.
Commissioning programme significantly extended.
Building fabric appears to perform less well than intended (U-values) and is no better than Part L 2013 standard.
MEV correctly commissioned, trickle vents missing from patio doors.
Some initial performance issues with ASHP systems.
Planned handover scaled back, due to COVID-19, but included digital resources and videos.
Mixed resident views on the handover.
Occupation
Homes sold at market rates; no premium applied for low carbon features.
GH did not highlight the technologies in marketing and many residents were unaware of them prior to purchase and/or moving in.
Positive feedback on design and layout, space and affordability of the properties.
GD collected energy monitoring data from the homes in-use, this enabled them to address operational issues during occupation.
Good winter thermal comfort reported but some summer overheating.
Good indoor air quality generally reported.
Some residents reported insufficient DHW at times, integral water storage too small.
Some residents found it challenging to work the heating controls.
Many residents reported energy bills lower than expected. However, many also reported the service charge as higher than expected. Some do not appear to understand that part of their energy bill is within the monthly service charge.
Some residents have concerns about future service charge increases.
GH plan to trial the Zeroth system again on larger schemes, taking forward the lessons from Tallack Road.
1. ASHPs and a thermal store maintain the ambient loop at 15°C to 25°C for distribution, with the temperature stepped up within the dwelling.
2. The thermal line is the primary insulation layer. This should be designed to form a continuous layer around the building to avoid thermal bridges and maximise energy efficiency.
Part 2: Detailed project findings
Part 2 of the report comprises the following sections:
- Section 5 presents the findings from the social research of the drivers and barriers to the supply of low carbon homes
- Section 6 presents the findings from the social research of the drivers and barriers to the demand for low carbon homes
- Section 7 presents the technical analysis of delivery of the case study homes and achieving low carbon performance
- Section 8 considers broader findings from an energy systems perspective
- Section 9 provides an overall summary of the key findings
- Section 10 proposes activities arising from the key findings
5. Case study and wider industry views on drivers and barriers
The key learning from the case study observations and interviews, industry focus groups and wider technical interviews is summarised here in relation to the drivers, enablers, and barriers in the delivery of low cost, low carbon homes.
Drivers:
- Building Regulations compliance - All stakeholders said that to deliver the construction of low carbon homes at scale, national regulation is necessary to set a higher minimum performance standard
In advance of this, and potentially at a local or organisational level, other drivers apply:
- A local vision for low carbon homes and an increased awareness of climate emergency. This was a key driver for the case studies, and was typically led by the local authority, affordable housing provider or local community group. The performance targets to deliver low carbon homes were influenced by the local authority in all the case studies, either as a landowner or as local planning authority. Developing the local supply chain and benefiting the local economy was also a driver in one case study
- Local planning policy. Local planning authorities had set ambitious carbon targets to respond to specific local conditions such as infrastructure capacity or land values
- To deliver lower running costs and improved comfort. This was stated as a benefit of low carbon homes by all supply-side case study stakeholders
- To support brand and product development to address low or zero carbon targets. Three out of 4 case study developers positioned themselves in the market to address the increased awareness of climate change and associated demand for low carbon homes amongst the general public, funders, and local authorities
Enablers:
- Collaboration with low carbon stakeholders. In some cases, expert advisors, either organisations or individuals, were driving the low carbon homes
- Grant funding for low carbon technologies or advice. Public grant funding for technologies was received by 2 of the case studies: Active Homes Neath and Etopia Homes. The future residents of Marmalade Lane received support to develop their housing brief which included a low carbon specification
- Investment by manufacturers in low carbon technologies. Private investment by manufacturers drove the construction technologies on 3 of the case studies
- Increased demand for real estate investments that meet Environmental, Social and Governance (ESG) funding criteria
Barriers:
- The lack of certainty about future requirements for 2025 and beyond was identified as both a barrier and a driver by stakeholders
- The perception of increased capital cost and challenging market conditions. Developers of low carbon homes said they lose bids for land because they have additional delivery costs compared with rivals targeting poorer carbon standards. Housebuilders said this additional cost is a key reason for them not building low carbon homes. Case studies demonstrated there was an increased cost, but this could be reduced through leaner design and business decisions, so that it does not adversely affect viability. Some case studies were delivered to a cost below stakeholder expectations that could be further reduced if delivered at scale
- Construction industry inertia. Stakeholders reported a reluctance within the industry to change supply chains and improve designs. Contributing factors included insufficient low carbon supply chains, lack of confidence in innovative technology, fear of reputational damage or claims, lack of knowledge-sharing across the industry, and warranty/standards issues with some products, such as MMC products. There is also a lack in diversity of offering (self-build sites, co-housing sites etc.) and limited interest in the long-term performance of homes that are sold
- Innovative products and a mismatch of knowledge and skills across the industry. This combination affected the performance of the case study homes and was cited as a barrier by stakeholders. Research team observations of the case studies clarified that this did not prevent the homes from being specified to be low carbon, but it significantly affected the design co-ordination, performance, and cost (especially the running costs)
- Insufficient supporting energy infrastructure. The issues raised included the lack of capacity in local electricity grids for fully electric homes with upgrade costs being borne by developers, despite them not owning or having guaranteed access to the upgraded infrastructure;
- Concerns about negative consumer attitudes to innovative technologies and homes. Stakeholders reported a range of potential issues which may be barriers to consumer demand for low carbon homes, including complexity of operation, lack of consumer understanding, and the risk of overheating. Research has confirmed this is a serious consumer concern, see Section 6
5.1 Introduction
The drivers and barriers to the supply of low cost, low carbon homes were identified during interviews with 56 stakeholders across the 4 case studies, encompassing developers, designers, and contractors, between 2018 and 2021. The themes were further analysed with 3 separate focus groups with members of the property, construction, and energy industries throughout 2020 and 2021. In addition, in August and September 2021, 31 targeted interviews were carried out with wider technical stakeholders across all sectors relating to housebuilding. The interviewees were selected to capture views from all the major stakeholder groups.
5.2 Drivers
The importance placed on different drivers was strongly influenced by who owned the land and who was driving the brief. Local authorities and social landlords placed particular emphasis on addressing fuel poverty, prioritising lower running costs and low environmental impact. Private developers placed greater emphasis on the increased awareness of the climate emergency and the need to improve Building Regulations to drive up performance standards across the board. For developers and the wider construction sector, the main drivers for low carbon homes were:
Building Regulations
All stakeholders said only national regulation can set the minimum performance standard required to drive the construction of low carbon homes at scale. This message was reinforced by our literature review which recognised improved national regulation as the key driver.[footnote 24] Low carbon technology currently comes at a cost premium and there is a need for more design input to low carbon homes. For a developer to maximise profits, it will typically build to the minimum carbon standards allowed by national Building Regulations (and local planning policy).
In general, housebuilders perceive they have little incentive in voluntarily developing homes that exceed required standards. Risks included non-traditional specifications, weaker supply chains supporting innovative aspects, the additional time required (for design and construction), and extra costs. The exception is pilot scale research and development (R&D) projects. These R&D projects typically have grant funding to overcome additional costs and strong support from stakeholders in decision-making roles, such as the local authority or development funders.
For those who do wish to deliver homes with low carbon performance exceeding Building Regulations requirements, there is a risk of commercial disadvantage. Instead, they would like the regulations raised so they compete equally with other organisations.
If you have a level playing field and you do it in a proportionate way and a way that has got a visible horizon everyone adapts, everyone is working off the same level playing field and 5 years down the line it is fine. So we want to do the right thing but we need regulation to help us do it.
(Large housebuilder)
Some stakeholders discussed the importance of the Energy Performance Certificate (EPC), and how this can drive the market. One developer stakeholder stated that EPCs were key to investment decisions, and so should better reflect the performance of the homes.
They (investors) have no idea about the detail of it, but they’re just interested in if it’s got a good EPC rating and, you know, if we’re setting targets.
(Large house builder)
Another stakeholder suggested increasing demand by changing the new build range of EPCs to be from A to E rated:
If you want to change it (demand) easily, promote to our clients […] the energy efficiencies, you know, you’re an A rated to E rated and then see how we change this system where we have to.
(SME housebuilder)
Planning policy
A role for planning departments in standard setting was also identified by some stakeholders who wanted flexibility. For example, to allow local planning authorities to set more ambitious carbon targets and/or to respond to specific local conditions such as infrastructure capacity or land and property prices.
The Building Regulations are often after the whole thing’s been approved and it might be too late at that point to drive down carbon emissions and I think it does need to be dealt with upfront and planning definitely has that role.
(Planning officer)
Every local authority should have a climate change planner, a planner whose job it is to deliver on climate action and why that’s important is that it provides capacity to get into the nuts and bolts of these applications.
(Professional institute of planners)
I think we need to be more ambitious, we need to set higher standards for planning […] and I think planning (departments) can do a lot more, should do a lot more.
(MMC housing developer)
However, others, particularly larger developers, preferred the ‘certainty’ and national consistency of having low carbon requirements set within the Building Regulations only, with no locally-stipulated variations.
It seems absolutely ludicrous that we should have local variants in climate response from the carbon point of view. You know, this is a global emergency, it’s not worse in St Albans than in Newcastle, you know.
(Large housebuilder)
In addition to regulations and planning policy, the following drivers were noted:
Delivering lower running costs and improved comfort
This was stated as a benefit of low carbon homes by all supply-side case study stakeholders. The developers of private sale homes said low energy bills and improved comfort were a benefit of low carbon homes, and 2 out of the 3 case studies led by private developers marketed this aspect to prospective homebuyers. Three of the case studies aimed to deliver homes with reduced running costs, with only one developer not making this is a key aim. Low running costs were particularly important to the affordable housing providers, who stated that reducing fuel poverty was a key driver for delivering their low carbon homes.
We’re offering social homes here for people who are on low income and there is a maximum rental or sum of money that we can expect them to be able to afford, otherwise they just go into arrears or they don’t pay rent, so that all has to be balanced out, but increasingly the home of the future, well, the home of the future is greener, it certainly doesn’t result in carbon emissions in the way that current homes do, so that’s a tick, which is better for air quality, energy use, etc, etc, healthier.
(Large social housing developer)
Local vision for low carbon homes and increased awareness of climate emergency
This driver was typically led by the local authority, affordable housing provider, or local community group. The low carbon performance targets were influenced by the local authority in all of the case studies. In 3 cases, Active Homes, Etopia Homes and Marmalade Lane, the local authority was the original landowner, setting the brief for low carbon homes on these sites. The local authority was the key stakeholder from the start, helping to agree stretch targets to meet local low carbon aspirations. At Tallack Road, the local authority was not the landowner, but was able to set higher targets as planning authority. In this case the Greater London Authority (GLA) had set the low carbon targets at an improvement of 35% compared to Part L 2013 of the Building Regulations, and 20% renewable energy contribution.
In the absence of higher regulatory standards, a local vision focused on the benefits for individual residents and the wider locality, is key. Local authorities are responding to climate change concerns of councillors and voters, and some also recognise the opportunity for local green employment and investment associated with low carbon homes. However, national housebuilders aiming to deliver the same designs across all of their developments are not in favour of localised requirements.
Research identified a high level of awareness around the climate emergency, compared to the start of the project, and literature review in 2019. This increased awareness – at an organisational and personal level in funders, clients, design teams and on site – was evident in the case studies and the wider technical interviews, and in the general call for an improved carbon standard for housing. It is not confined to local authorities who can react through planning policy or by setting higher standards for their own developments. Leading developers and some SMEs have made their own commitments in relation to climate change and are acting accordingly, while some investment funds are looking to target their investments in this area.
I like thinking of it as a series of snooker balls. If the lenders, investors into our funds, have to demonstrate to their ultimate lenders that they are investing in environmentally efficient properties, then they put pressure on us, and we put pressure on our developers, who put pressure on the housebuilders. So yeah, I think that’s the dynamic.
(Investment director)
Brand and product development to address low or zero carbon targets
Three out of 4 case study developers positioned themselves in the market to address the increased awareness of climate change amongst the general public, funders and local authorities.
In the wider interviews, some stakeholders pointed towards the market opportunities of delivering low carbon homes and the need to adapt their brand to address this. Many stakeholders recognised the need for housebuilders to address the upcoming policy changes. Major housebuilders said they wanted to ‘do the right thing’ but cannot as their business model and the market requires them to deliver to the minimum standard. If they target delivery which is better than Building Regulations, and not driven by local planning conditions, they are less likely to win the development site via a competitive bid, as their costs will have increased without a sales uplift. This means that, together with sharing experiences and ensuring low carbon homes perform well and are liked by residents, eliminating the increased delivery costs is essential.
5.3 Enablers
The following aspects contributed to the delivery of the case study schemes but they are not necessarily a key part of upscaling delivery of low carbon homes.
Collaboration with low carbon stakeholders
In some cases, expert advisors, either organisations or individuals, were driving the delivery of the low carbon homes. Active Homes had an innovation partner involved early on driving an ‘active building’ approach. Etopia Homes had a consortium of low carbon organisations, including low carbon consultants, who were driving innovation in the brief and design. The developer at Tallack Road had low carbon expertise in-house and so responded to the local planning requirements by proposing an innovative low carbon solution. Expert low carbon advice, whether in-house or external – and sharing experiences, lessons learned - are key enablers for those new to delivering low carbon homes.
Grant funding for low carbon technologies or advice
Two case studies - Active Homes and Etopia Homes – won public grant funding for innovative technologies, which influenced the specification at these 2 case studies. Both developers learned from these grant funded schemes and created designs for the next iteration which were not financially supported in the same way. For Etopia Homes, the later units within the development did not have any grant funding, and some of the technologies - deemed not to deliver valuable carbon savings - were dropped. Pobl also refined its designs and while it continued to attract grant funding, the contribution level required was reduced.
The Marmalade Lane team received early-stage seed funding from the local council to provide professional consultancy to advise on the low carbon performance and community-led housing. It would most likely have benefited from this engagement continuing throughout the construction phase.
Investment by manufacturers in low carbon technologies
Three case studies adopted specific products as their main construction system. For Marmalade Lane and Etopia Homes, this contributed to the project finances. At Active Homes, this was driven by a desire to support research activity and local industry.
Increased demand for real estate investments that meet Environmental, Social and Governance (ESG) funding criteria
This was cited by stakeholders as a more recent (2021 onwards) enabler for low carbon housing and is supported by changes to the Sustainable Finance Disclosure Regulations. All wider stakeholders working in real estate investment and development stated that there was now (2021) much more finance available to develop low carbon housing.
5.4 Barriers
The main barriers for low carbon homes perceived by developers and the wider construction sector are set out below.
Lack of certainty about future requirements for 2025 and beyond
This was identified as a barrier as well as a potential driver by stakeholders. While this was feedback from the social research, the evidence from case studies highlights that the lack of a policy driver is not stopping a small part of the housing industry in voluntarily supplying low carbon homes.
Stakeholders reported that current regulations are not driving holistic low carbon designs, or addressing embodied carbon, building performance in use, post-occupancy engagement activities and simplicity for users. All of these factors contribute to a low carbon home which is low in operational and embodied carbon – in line with the UK’s net zero target for 2050. Part L of the Building Regulations only addresses carbon from regulated operational energy, via compliance modelling at the design stage, updated at the as-built stage.
In some cases, stakeholders reported that the Building Regulations and accompanying tools should be reviewed and updated to reflect newer technologies and approaches, such as MMC or innovative renewable heating technology that could not be modelled in SAP. This would allow the full range of approaches to be easily considered when determining strategy and demonstrating regulatory compliance.
There was also consensus in stakeholder interviews about the need for significant notice of details of changes to regulations, including a clear, detailed, far-sighted roadmap. This is to allow time for developers and the supply chain to develop and test solutions and build up their capability and capacity to deliver. There is the need for certainty regarding any new regulation, de-risking the chances of meeting future low carbon standards. Developers are planning developments now whose delivery timetables stretch into the 2030s and beyond. Understandably, they want to know about substantive changes in regulations that will impact on these long-term projects, such as the scope and the level of future carbon and energy targets. This will then be reflected in their design responses relating to energy supply, construction type and design integration. If they need to change current designs, they want the new approaches to have some longevity.
2025 is tomorrow for us. You know, I’m looking at proposed developments which are going in for planning now (2021) or we’re about to submit to planning, where the first legal completions are going to be in 2024 and they’ll be running through to 2025, 6, 7, 8, years beyond. So, we’re actually designing the schemes now (2021) that we’re going to have to deliver to those regulations (2030), we’re designing them now and we don’t know what the regulations are going to be.
(Large housebuilder)
There are (extra) costs involved (for low carbon homes), but if it’s a level playing field, then it’s just kind of, I don’t know, it’s not such a big thing.
(Large housebuilder)
Challenging market conditions and land economy factors
Developers of low carbon homes say they lose bids for land due to the additional delivery costs, where they have to compete with the wider market targeting worse carbon standards.
We would love to be building low carbon homes now, but we can’t afford to do it because [large house builder X, Y and Z] would buy every bit of land we bid for.
(Large housebuilder)
Increased capital cost and poorer scheme viability
For example, additional capital costs, design costs, and/or utility infrastructure costs). There were mixed views across the stakeholders on the significance of extra construction cost as a barrier. Some stakeholders said that additional capital cost was not a barrier to low carbon homes, as these homes were being delivered within a normal range of construction costs and were viable at scale. However, most stakeholders perceived a lack of financial incentives to deliver low carbon homes.
I would say that we’ll pay anything up to about a 5% premium in build costs. For apartments we’re actually able to build Passivhaus apartments at 3% cheaper than BCIS (Building Cost Information Service) Building Regs compliant equivalent.
(Local Authority housing developer)
Insufficient supporting energy infrastructure
The issues raised included the lack of capacity in the local electricity grids for fully electric homes and upgrade costs being borne by developers, despite them not owning or having guaranteed access to the upgraded infrastructure.
Economically it’s a problem because, as I said, everything would be electric, so we need the utility providers, the DNO[footnote 25] or UKPN[footnote 26] or whatever needs to give us all this capacity to install, because we need it, because otherwise we cannot install, we cannot meet all their requirements. (Construction site manager)
Every time I go and build a new development in London on a brownfield site, I always, for some reason, have to upgrade the infrastructure and pay UK Power Networks a huge amount of money.
(Large housing association)
The problem with infrastructure or electricity upgrades is that you could upgrade it and then it’s not necessarily yours. So you could upgrade the substation to meet your requirements and then the developer next door could come in 5 years later but take the electricity first […] so it becomes a high cost and high risk.
(Large housebuilder)
Construction industry inertia
Stakeholders reported a reluctance within industry to change supply chains and designs. Contributing factors included insufficient low carbon supply chains, lack of confidence in innovative technology, fear of reputational damage or claims, lack of knowledge sharing across the industry, and warranty/standards issues with some products such as MMC products. This also links to the point above about wanting certainty about future requirements prior to making changes.
I sense a real resistance to [change], developers are so used to doing things the way they do.
(Local authority housing developer)
Anything that you haven’t done before is always a risk. Whether the piece of machinery in itself is a risk, or the installation of it is a risk, or the specification of it is a risk, or the operation of it by the customer.
(Large housebuilder)
The research highlighted a lack of diversity of offering in the housing market and limited long-term interest in performance. Stakeholders raised concerns about a lack of diversity in the housing market, with the market being dominated by the major housebuilders and with few custom build sites and few sites where developers retained a long-term interest in the performance of the homes.
The development industry in the UK is dominated by a small number of relatively large national firms with the fundamental economic characteristic of any oligopoly, which is very low innovation, lack of diversity and poor amount of competition.
(SME housing developer)
Innovative technologies and mismatch of knowledge and skills across the industry
These 2 factors affected the performance of homes and were cited as a perceived barrier by stakeholders. Case study observations clarified that this did not prevent homes from being specified as low carbon, but it significantly affected the design co-ordination, performance, and cost (especially the running costs).
The report identified knowledge gaps across development teams. A holistic understanding of low carbon homes was missing. Teams were unaware of the potential impact of their decisions or of the cost-saving potential of a fabric-first approach. There was a lack of knowledge and experience on the part of clients, commercial teams (quantity surveyor [QS], buyers and sales teams), planners and funders, whose early-stage input drove sub-optimal outcomes in the case studies.
I think designers and technical staff, in-house builders, procurement teams, site managers, people on the sales side certainly need upskilling to understand the technology and to be able to explain and communicate properly to consumers how they should use the system in an optimal way […] because there is no mass market understanding of these technologies at the moment.
(Trade association for house builders)
We have had a very, very strong message from our members that people really do need support. They need guidance about how to do things and what works and what doesn’t work.
(Trade association for house builders)
On site, there was a mixed level of skilled labour and training provision across the case studies, with some schemes showing best practice installation and demonstrating ‘on the job’ site training in relation to MMC and innovative low carbon technologies. All the case studies had expert knowledge on site in charge of installing the low carbon technology, and a bespoke system of training other specialists on the job. Wider stakeholders cited shortages of skilled commissioning engineers, performance evaluators, building control and maintenance staff.
You find that the person who is supervising the work on site doesn’t really know what it is they’re supposed to be presenting or achieving. It sounds absolutely crazy and frightening, but it’s out there and you know, it’s not a rare case.
(Building Control team leader)
The research team witnessed a lack of understanding of certain technologies on site, electrical demand management, a lack of integration between technologies, and poor co-ordination between architectural elements and building services. Supply chain issues and lack of knowledge was also cited by stakeholders as a challenge to delivery of low carbon homes. Where innovative products were used, there were specific practical issues on site, resulting in poorer performance and longer completion times.
There’s a lack of understanding about demand management and storage at this point in time […] so I think that’s why [electrical demand] becomes prohibitive, because it’s done to that [SAP] calculation rather than the reality of the actual usage on the schemes.
(Large house builder)
Concerns about negative consumer attitudes to innovative technologies and homes
Stakeholders reported a range of potential issues which may be barriers to consumer demand for low carbon homes, including complexity of operation, lack of consumer understanding, and the risk of overheating. The research has confirmed this is a consumer concern, see Section 6. Related to these risks are perceptions of consumer preferences for familiar technologies, and of a lack of market demand.
We have just completed an interesting piece of market research about what the consumers understand by zero carbon and Passivhaus, only to understand they don’t understand anything, other than it’s a good thing and it ticks a box and makes them feel warm and fuzzy.
(Legacy landowner)
6. Residents and consumer views on drivers and barriers
The key learnings from the research with case study residents and wider consumer survey with 2,500 respondents are summarised here in relation to the drivers, barriers, challenges, and benefits of living in sustainable homes.
Drivers:
The low carbon aspects of a property are not a principal driver when purchasing a home. The social research with case study residents found the main factors were location; size and space; and the design and layout of the home. Affordability was also perceived to be a key factor for most residents in their decision-making process. The key drivers were:
- Lower energy bills: Lower energy costs was cited by many of the case study residents, with some taking into consideration the perceived higher upfront cost of their properties with the potential for long-term energy bills savings
- Reduced environmental impact: Having a more sustainable home and reducing their personal environmental impact, in addition to saving money on energy bills, was a driver for many of the case study residents. In the wider consumer survey, many respondents were attracted by the prospect of both using a renewable source of energy and being environmentally responsible, and 11% would be willing to pay more for a sustainable home
- Warmth, comfort and good air quality: This was a key driver for most case study residents and an expected benefit for 41% of wider consumer survey respondents. Residents expect low carbon homes to maintain a steady temperature throughout the year due to the high levels of insulation
- New, modern and high-quality properties: Many of the case study residents were attracted to the design, layout and quality of the properties. In addition, a new home warranty was reassuring
- No gas in the properties: All case study schemes were electrically heated and had no gas, with some residents believing this made their homes safer as well as more environmentally friendly. Whether a home used gas or electricity for heating and cooking had little impact on the views of respondents in the wider consumer survey
Barriers:
The main perceived barriers to purchasing and renting and living in low carbon homes were:
- Perceived higher cost of low carbon homes: Although case study properties were sold at market rate, around half of their residents believed low carbon homes were more expensive than traditional homes. In the wider consumer survey, 61% of respondents saw cost as a main barrier to buying or renting a sustainable home;
- Lack of awareness or availability of low carbon homes: In the wider consumer survey, 37% said location was a main barrier to purchasing or renting a sustainable home, and 35% stated there was a lack of availability. Very few case study residents were aware of other similar developments. They said information about low carbon homes was generally lacking
- Concerns about performance and reliability: Some case study residents were concerned developers and workforces delivering low carbon homes lacked the knowledge and experience to get everything right. Of the 238 respondents from the wider consumer survey who said they would not consider living in a sustainable home in future, 36% said it was because they were worried about things going wrong, and 27% said they were concerned about technology in the homes
- Concerns about interior space and the visibility of technology: Some case study residents were concerned that the technology, wiring, and pipework etc, would be very visible and take up space in the home. In the wider consumer survey, 59% said they would be happy to live in a sustainable home that looked different from a typical home; 12% said it would deter them; and 29% said it would depend on how it looked different
- Servicing and repairing low carbon technologies: Some case study residents shared maintenance concerns about the low carbon technologies, suggesting few workers would have experience with them. It was also a key concern for respondents of the wider consumer survey, with 39% citing maintenance as a key challenge of living in such a home. As such homes and technologies become mainstream, these concerns and potential issues are likely to reduce, especially if the supply of low carbon homes is driven by Building Regulations
- Potential delays to completion and snagging issues were key concerns raised by some case study residents in pre-occupancy interviews. They expected low carbon homes would take longer to build and have a higher chance of delays during construction. This would be a barrier for some potential residents, especially if they did not have the flexibility to endure such delays. Some also raised concerns about snagging issues, and expected more problems with their new homes than with more traditional homes
Challenges:
The key challenges experienced by the case study residents living in low carbon homes were:
- Learning about and using the technologies: Most case study residents, and 31% of the wider consumer survey respondents, expected difficulty understanding and using unfamiliar technologies and control systems - and with their servicing and repair. In practice, in each case study, while some found it relatively easy, many residents struggled with them. Some had no wish to interact, expecting that they would not need to, as they believed the systems would be largely pre-set and automated
- Having insufficient domestic hot water: This was a concern for a few residents prior to moving into their new homes. Once living in a low carbon home, some residents in 3 of the case study developments reported having insufficient hot water
- Actual energy bills: Case study residents expected their low carbon homes to have low energy bills. However, a low carbon design does not necessarily translate to low energy bills, especially when moving from gas heating to an electric heat pump, due to the significantly different energy unit costs. Many case study residents were receiving and paying energy bills that were higher than expected (sometimes significantly higher)
- Constraints on behaviour: Although not expected prior to occupancy, around half of case study residents felt that their homes in some way imposed constraints that required changes to their behaviour, and this was a challenge. This was something they would like to have been advised about and understood prior to moving in
Benefits:
The main benefits to living in low carbon homes identified by the case study residents were:
- Lower energy bills: Some case study residents did receive the lower energy bills they expected. This was not the case for many of them, though
- A warm and comfortable internal environment: Most case study residents reported that homes were comfortable and retained heat well, a bonus for the winter months. Some experienced overheating issues during the summer, but this generally improved as they got used to using their systems, implemented solar shading solutions and/or window-opening routines
- Good air quality and ventilation: Most case study residents reported that the air quality in their homes was good and that there was good air circulation. This was considered to be a key benefit of the properties as none experienced significant issues with mould, damp, or condensation
6.1 Introduction
The drivers and barriers to the purchase or rent of low cost, low carbon homes, and the experiences of living in such homes were identified through detailed interviews, focus groups, and diaries from 55 householders from across the 4 case studies. These findings were analysed together with the views expressed via the wider consumer survey of 2,500 participants. In combination this feedback comprises views from actual or potential buyers, renters, and occupiers.
6.2 Overview
The low carbon credentials of a property are not a principal driver when purchasing a home. Social research with case study residents found the main factors influencing their choice of home were location; property size and space; and the design and layout of the home. Cost and affordability were also key factors for most residents, considering all aspects of a property in relation to its financial cost and value for money. Most case study residents demonstrated good awareness of sustainability and environmental issues, and many considered sustainability and energy efficiency in their decision-making process, but these were not the main factors influencing their choice of home.
Other studies have revealed a similar outlook. In 2012, the NHBC Foundation[footnote 27] held interviews and focus groups with residents and found location was the key consideration when purchasing a new home. Although low carbon homes helped residents save money on their energy bills, energy efficiency remained a minor consideration. However, more recently in 2018, the NHBC Foundation[footnote 28] assessed the priorities of different categories of buyers via a large-scale national survey. It suggested older downsizers, and those in higher density urban settings, place greater emphasis on energy efficiency, but it remains a relatively low priority for other groups.
The wider consumer survey undertaken as part of the Building for 2050 project showed the following:
- of those who have experience of being in a sustainable home (circa 14% of all respondents[footnote 29]), 89% described it positively and only 2% reported a somewhat or very negative experience
- 67% of all respondents to the wider survey would consider living in a sustainable home, 23% did not know, and the remaining 10% would not consider living in one. Of the 10% (238 respondents) who would not consider a sustainable home, their main reasons were; the cost of upkeep (52% of respondents); concerns that things will go wrong (36%); the initial cost of purchase and renting (34%); and concerns about the technology (27%)
- 76% of respondents who have heard of sustainable homes would consider living in one. Of those who had not heard of sustainable homes prior to the survey, 60% said they would consider living in one after they were provided with a definition. Furthermore, 11% of all respondents said they would be willing to pay more for a sustainable home
Respondents were asked if they had heard of a sustainable home and then what they thought a sustainable home was. After this, the research team provided a broad definition: “Some homes are now being built which are designed to be better for the environment, both in how they are built and the amount of energy needed to make them comfortable to live in. Some refer to these dwellings as sustainable homes.” An image was shared alongside the definition as an example, but it was noted that a home does not need to have all of the features shown to be considered sustainable.
During the wider consumer survey, respondents were shown pairs of houses (one with traditional attributes and the other with sustainable attributes) and asked to select their preferred option. The initial price to purchase or rent was a key factor, with most saying they would choose to live in the cheapest home, whether sustainable or traditional. However, when the cost was the same for the 2 types of property, around 85% (although this varied slightly depending on sustainable aspects of the home) said they would choose the sustainable home (assuming it would deliver at least 25% savings on energy bills). The proportion reduced to around 45% if the sustainable home was more expensive to purchase and rent, even with up to 50% saving on energy costs.
6.3 Drivers
The project has identified the main drivers for residents choosing to buy and/or live-in low carbon homes, with Figure 7 showing these across the 4 case studies.
Figure 7: Main drivers for case study residents
Description of Figure 7:
Chart showing the main drivers for case study residents (from high to low).
- Location (Pre-design factor)
- Interior and exterior design and layout (Design)
- Property size and space (indoor and outdoor) (Design)
- Financial cost/affordability of home (Procurement)
- Sustainability/energy efficiency (Procurement)
- Low energy bills (Procurement)
- New/modern building/good quality/having a guarantee (Procurement)
- Warm, clean, and comfortable home (Procurement)
- No gas at the developments and homes (Construction)
- Garage and parking space (Construction)
The summary below integrates the results from the wider consumer survey and the case study interviews. It is interesting to note that cost and value were among the principal drivers. Other than location, property size and space, and the purchase price of properties, the key drivers related to the low carbon aspects were:
Lower energy bills:
This was a key driver for some of the case study residents, with some taking into consideration the perceived higher upfront cost of their properties with the potential for long-term energy bills savings.
The lower environmental impact of the property:
Many of the case study residents were attracted by the prospect of lower energy usage. Some residents explained they were seeking to lower their environmental impact, but most said their interest was driven by the prospect of lower energy bills. The wider survey highlighted how many respondents were attracted by the prospect of both using a renewable source of energy and being environmentally responsible. In addition, in the wider consumer survey, when selecting between pairs of houses, 44% always chose the sustainable home regardless of cost (assuming at least 50% saving in energy bills), 14% always chose the traditional home, and 42% sometimes selected sustainable homes and other times selected traditional, depending on cost and other attributes. When asked about benefits and challenges of sustainable homes, 58% expected lower energy bills to be a benefit and 13% expected higher energy bills to be a challenge.
Warmth, comfort, and good air quality were rated highly in the wider consumer survey:
The case study residents expected this to be a key benefit from living in their new homes, and this was generally realised in practice, particularly during winter months. Further details are provided in Section 4.2.3.
New, modern, and high-quality properties:
While the size and space in a property were among the most influential drivers, many respondents were attracted to the design, layout, and quality of the properties. They appreciated the aesthetics internally and externally, and felt the design suited their needs well. Having a new home was a key driver for some, and many they felt reassured and comforted about their decision to buy a sustainable home with a new home warranty.
No gas in the properties:
Some residents believed this would make homes safer as well as more environmentally friendly. Prior to occupancy, a small number of case study residents did have concerns about how well their home would perform with only electricity for heating and cooking (after many only previously used gas), but all were generally satisfied with these aspects. Although some felt gas was more powerful and easier to control (mainly in relation to cooking), they expected they would get used to it over time and still felt safer not having a domestic gas supply. In the wider consumer survey, whether a home had gas or electric heating had little impact on respondents’ views. Similarly, a change in cooking fuel from gas to electricity or vice versa did not impact on which of the 2 choices of home was preferred.
6.4 Barriers
The project has also identified the main barriers for residents when choosing to buy or live in low carbon homes, with the main barriers perceived by case study residents shown in Figure 8.
Figure 8: Main barriers perceived by case study residents
Description of Figure 8:
Chart showing the main barriers perceived by case study residents (from high to low).
- Concerns about performance/reliability of homes and technology (Pre-design factors)
- Learning about new technology and systems and how to use them (Design)
- Concerns about potential delays in construction of new low carbon homes (Design)
- Uncertainty with energy bills/service charge (Procurement)
- Concerns about internal comfort in the homes (Procurement)
- Concern about space occupied by low carbon technology in the homes (Procurement)
- No gas at the developments and homes (Construction)
- Not having a combi-boiler/concerns about hot water (Construction)
The points below integrate the results from the wider consumer survey (see Figure 9) and the case study interviews.
The perceived higher cost of low carbon homes:
As highlighted in the wider consumer survey, the main barrier was the perception that low carbon homes would be more expensive than more traditional homes, with 61% of respondents perceiving this to be the main barrier.
For case study schemes, sales prices were set by the average sales price in the area, but around half of their residents thought sustainable homes were more expensive than traditional homes - even though they did not feel this was the case with the homes they had purchased.
Property cost was the main driver in the wider consumer survey, as respondents were shown pairs of houses and asked to select which option they would choose to buy or rent, with each pair consisting of a sustainable home and a more traditional home. When the option of a sustainable home was shown to respondents, between 85% and 88% (depending on sustainable attributes included) selected the sustainable home when the property cost was the same as the traditional. This reduced to 40-42% when the property cost of the sustainable home was 20% higher.
In the wider consumer survey, when those who would consider living in a sustainable home (1681 respondents) were asked about their willingness to pay more:
- 58% said they wanted an environmentally friendly home, but most said they would not or could not pay more for it
- 16% of those who would consider living in a sustainable home are willing to pay more (11% of all respondents)
The wider consumer survey also highlighted concerns about the ability to obtain mortgages and the resale value of sustainable homes. A negative impact on resale value was also an issue raised by some case study residents, particularly regarding the performance of the low carbon technologies installed.
Lack of awareness or availability:
Lack of awareness or availability of low carbon homes in the locations where people are looking to live is another key barrier evidenced by the wider consumer survey. A further barrier is a lack of awareness and information of low carbon homes in locations of interest. In short, how do house-buyers find out about them?
Many of the case study residents felt there is a growing demand for sustainable homes, but very few were aware of other developments with sustainable homes and felt that such homes were largely confined to those who choose to build their own properties.
Concerns about the performance and reliability of low carbon homes and technology:
Some case study residents discussed how they lacked confidence in whether the developers and the workforce delivering the low carbon homes had sufficient knowledge and experience to get everything right. The choice to purchase a low carbon home was perceived by some case study residents as a ‘risk’, especially if buying off-plan. This included doubts over the ability of developers and workers to resolve any performance issues. Many respondents felt that more data and evidence should be provided to prove the performance of such properties (for example, energy bills for a show home for a specific period) to help reassure them about their purchasing decision.
Many case study residents had concerns about the low carbon technologies in their homes as they only had experience using conventional systems, citing it as a key barrier.
Most case study residents were hoping and expecting to receive comprehensive information about their homes and systems as well as receiving a demonstration in their new homes. They felt that the delivery had fallen short of their expectations, but many did acknowledge that COVID-19 likely had a significant impact on the amount and quality of training and information provided.
Potential delays to completion and snagging issues:
These were among the key concerns raised by some case study residents in pre-occupancy interviews. They expected low carbon homes would take longer to build and have a higher chance of delays due to issues during construction. They felt this would be a barrier for some residents, especially if they did not have the flexibility to endure such delays. Some worried about potential snagging issues and expected more issues with their homes compared with more traditional homes.
Concerns about interior space and the visibility of technology:
Concerns about interior space and the visibility of technology were highlighted by some case study residents as potential barriers prior to occupancy. They were concerned that their homes would have visible technology, wiring and pipework that would take up too much space. Although visibility was not an issue during occupancy, some residents did report a lack of storage space (particularly at Marmalade Lane and Active Homes). The size and location of low carbon features impacted on this and reduced their accessibility to certain areas of their homes (for example, Active Homes’ residents having no access to their attic spaces where the ventilation unit and ductwork were located).
Appearance:
More generally, the wider consumer survey investigated whether the aesthetics of the property created a barrier to purchase or rent. The findings showed that 59% of consumers would be happy to accept sustainable homes looking a little different to a typical home; 12% said it would deter them or that a property needs to look like a typical home; and 29% said it would depend on how it looked different.
Figure 9: Main barriers to purchasing or renting a sustainable home from the wider consumer survey (%, Base = 2500)
Barriers | % |
---|---|
Cost of the property | 61 |
Location of these homes | 37 |
Lack of availability of properties | 35 |
Lack of information and publicity | 28 |
Concerns about potential resale of the property | 25 |
Inability to secure a mortgage / funding | 23 |
Having something new or unknown | 20 |
Having something different to others | 10 |
No barriers in particular | 7 |
6.5 Challenges
This research has also highlighted the main challenges expected or experienced living in low carbon homes (Figure 10). It is important to highlight that some of the problems faced by residents were teething issues - each of the homes had one or more elements that were innovative.
Figure 10: Main expected challenges for case study residents
Description of Figure 10:
Chart showing the main expected challenges for case study residents (from high to low).
- Concerns about performance/reliability of homes and technology (Pre-design factors)
- Quality of training and information provided about the home (Design)
- Learning about new technology and systems and how to use them (Design)
- Internal comfort in the homes (particularly overheating) (Procurement)
- Air quality/performance of ventilation systems (Procurement)
- More snagging issues expected than with a more traditional home (Procurement)
- Getting accustomed to new home and living space (Procurement)
- Supply and delivery of hot water (Construction)
- Maintenance of technology/systems (Construction)
- Limited availability of modifying the homes (Construction)
- Adjusting to not having gas (Construction)
- Uncertainty with energy bills/service charge (Construction)
This summary, which integrates results from the wider consumer survey (see Figure 11) with findings from the case study interviews, identifies the following key challenges:
Servicing and repairing low carbon technologies:
For 39% of survey respondents, finding someone to fix things if they go wrong, and maintenance of the technologies, were perceived as the main challenges to living in a sustainable home.
The case study homes are new, and the performance issues identified tended to be associated with installation – and therefore the responsibility of the developer – rather than maintenance issues. Active Homes attempted to address this issue by having the maintenance contractor for their property portfolio as the heating system installer as well. However, the benefits do not appear to have been fully realised yet, as the engineers that visited the properties to principally resolve early operational issues were reported by case study residents to have insufficient skills or knowledge to resolve some of the issues that arose (suggesting they may not have the same level of expertise as the installation team).
Learning about and using the technologies:
Prior to occupancy, most case study residents (and 31% of those from the survey) had concerns they would have difficulty understanding and using unfamiliar technologies and control systems. This was perceived to be a significant barrier and ongoing challenge.
In practice, in each case study, while some residents found it relatively easy to manage their systems, many struggled, and some had no wish to interact with the technology assuming it would be pre-set and automated. Residents at Etopia Homes and Active Homes found their systems to be more challenging to use due to the complexity of their M&E systems. There were fewer issues for residents at Tallack Road, which used a communal heating system with an in-dwelling heat pump in place of the usual boiler, and residents at Marmalade Lane were able to work together more closely as a co-housing community to help each other out.
While residents were provided with information on the low carbon features and how to operate them, they said there was a lack of training and information about how to optimise their systems and how they should be operated in conjunction with each other, as well as how to adjust systems to tailor them to their needs. Across all 4 case studies, most residents also commented about how they felt the information they were provided with was not user-friendly, with some only receiving installer guides for their systems. The COVID-19 pandemic put some limits on engagement however, and many of the developers had planned face-to-face training but felt they were not able to provide this due to the circumstances at the time. Most residents also acknowledged this, but some felt that more could and should have been provided, while taking appropriate Covid-related precautions. Due to the perceived lack of information and training, learning how to operate their homes and systems, and having confidence in them, became one of the main residential challenges. It suggests a need for comprehensive training to ensure homeowners know how to set up systems to deliver their requirements in an energy efficient way.
Residents said there was insufficient forethought to ensure the controls were simple to understand and operate (and thus minimise the time needed for training and the risk that the homes would not be operated effectively). Both Etopia Homes and Active Homes offered apps to help control the systems, but there were issues in both accessing and using these apps, as well as the general controllers in their homes (particularly using and programming heating systems). Some residents did not wish to use apps and were pleased to find there was an alternative control option. Some residents felt they would have preferred to have a single controller that could be used for all systems in the home rather than having to operate separate controllers for each system.
Across the 4 case studies, residents were more satisfied with homes that had simpler services designs (Marmalade Lane and Tallack Road vs Active Homes and Etopia Homes). This reflects a combination of more complex services strategies not working effectively and concerns of whether this would be addressed, and the ease of operation of such systems. The above findings demonstrate that the systems need to be suitable for a range of users. More fundamentally, the simpler the system is for the resident to operate and meet their needs, the easier handover will be.
It is important to highlight that some of the problems faced by the case study residents were teething issues which may not be representative of a wider roll-out of the low carbon designs - each of the homes had one or more elements that were innovative. This affected both the installed performance of the systems as well as the developers’ ability to help residents use them, due to being unfamiliar with the systems themselves. In some cases, this made some residents feel like ‘guinea pigs’ in an experiment, and some had concerns that the developer did not understand the technology and doubted the issues would be fully resolved. These residents recommended that the designs and technical systems need to be proven first before being installed in homes, and that evidence needs to be provided of the in-use performance of the systems.
While consumers will become familiar with new systems over time, some may consider purchasing or renting a low carbon home to be too big a risk until the homes and technologies are proven (36% of respondents in the wider consumer survey stated that they were worried things would go wrong, and 27% were concerned about technologies). However, developers will only gain sufficient experience when the first homes they build are lived in, potentially relying on early adopters who are willing to accept new technologies and pay more for a sustainable home, as evidenced by the results of the wider survey. These dwellings will need to be in locations where early adopters want to live.
Insufficient domestic hot water (DHW):
In 3 of the 4 case studies, some residents reported having insufficient hot water. Unlike a common form of DHW supply via a gas combination boiler, heat pumps do not provide instantaneous hot water, and require a hot water cylinder. Many regular gas boilers also rely on hot water cylinders, but they operate at a higher flow temperature than heat pumps, meaning that they can more quickly replenish hot water and the supply effectively goes further (less hot water to cold water is required for a comfortable temperature). The one case study where residents did not report problems had large hot water cylinders.
Actual energy bills:
The main benefit expected from low carbon homes by both case study residents prior to occupation, and survey respondents, was lower energy bills. There was an assumption they would be more energy efficient, leading to lower energy consumption and bills. Indeed, developers told residents in 3 case studies were that there would be low energy bills (not simply lower than current). However, a low carbon design does not necessarily translate to a low energy design. In particular, in moving from gas heating to an electric heat pump, the higher unit cost of fuel can outweigh the efficiency savings from adopting a heat pump. Such experience on a wider scale would negatively impact the public’s perception of low carbon homes.
Constraints on behaviour:
Around half of the case study residents recommended that any required changes in behaviour associated with living in a low carbon home should be clearly explained when people are considering purchasing or renting homes. For example, Etopia Homes set a maximum space heating temperature of 22°C, and Active Homes included window sensors to switch off the heating if a window or external door was open. In both cases, residents were unaware of the intervention. These operational issues faced by residents highlight the importance of providing clear information to prospective residents as well as a comprehensive handover when residents move in.
Figure 11: Main challenges to living in a sustainable home from the wider consumer survey (%, Base = 2500)
Challenges | % |
---|---|
Difficulties finding someone to fix things if go wrong | 39 |
Maintenance of the technology in the property | 39 |
Difficulty understanding and using the technologies | 31 |
Having a complicated home/high maintenance costs | 30 |
Having to change behaviour/habits in the property | 23 |
Difficulty controlling the temperature/comfort | 18 |
Less comfortable indoor environment in winter | 13 |
Higher energy bills | 13 |
Less comfortable indoor environment in summer | 12 |
Poor air quality/ventilation in the property | 11 |
Social stigma/having something different to others | 8 |
No challenges in particular | 12 |
6.6 Benefits
The main expected benefits for case study residents are shown in Figure 12.
Figure 12: Main expected benefits for case study residents
Description of Figure 12:
Chart showing the main expected benefits for case study residents (from high to low).
- Location (Pre-design factor)
- Interior and exterior design and layout of homes (Pre-design factor)
- Low energy bills (Pre-design factor)
- Property size and space (indoor and outdoor) (Design)
- Sustainability/reducing environmetal impact (Design)
- New/modern building/good quality/having a guarantee (Procurement)
- Warm, clean, and comfortable home (Procurement)
- Good air quality/ventilation systems (Procurement)
- Value for money/potential resale value (Procurement)
The wider consumer survey also asked respondents about expected benefits, see Figure 13.
The summary below captures the perceived benefits as well as the actual benefits delivered at the case study developments.
Lower energy bills:
The main expected benefit of respondents in the wider consumer survey was low or lower energy bills, and this was also a key benefit expected by case study residents. As noted above, for the case study residents, views regarding their energy bills were mixed, with some experiencing the lower bills they expected while others found them higher (sometimes significantly higher than they were paying in their previous homes, which they considered to be less energy efficient and of poorer quality).
Reduced environmental impact:
Having a more sustainable home and reducing personal environmental impact was, for some, a key benefit to living in such a home. Some residents explained how they considered it to be an ‘added bonus’ and appreciated how their carbon footprint could be reduced without having to significantly alter their behaviour. Others felt this would encourage and enable them to adopt more sustainable lifestyles and activities. Some residents discussed how they were proud to be moving into and living in a new low carbon home and hoped that their decision and experiences would have a positive impact on future properties of this type. All residents felt that more low carbon homes should be built.
A warm and comfortable environment:
Most case study residents reported that, as expected, their homes were comfortable and retained heat well, with some finding they maintained a steady and consistent temperature through the summer and winter periods. This was also identified as an expected benefit in the wider survey. Some case study residents experienced overheating in the summer, but this generally improved as they got used to using the technology in the homes or implemented solar shading solutions and window-opening routines.
Good air quality and ventilation:
This was identified as an expected benefit in the wider consumer survey. Most case study residents reported that the air quality in their homes was good, there was good air circulation, and they had not experienced any issues with mould, damp, or condensation. This was considered a significant benefit to some as they compared it to problems encountered in previous homes, and a few commented about how they felt their health had improved as a result of this in their new homes, particularly for those with respiratory issues.
Some of the other drivers, such as using a renewable source of energy, were also identified as benefits in the wider consumer survey, as shown in Figure 13.
Figure 13: Main benefits to living in a sustainable home from the wider consumer survey (%)
Benefits | % |
---|---|
Lower running costs (energy bills) |
58 |
Using a renewable source of energy | 51 |
Being environmentally responsible | 50 |
Warm and comfortable indoor environment in winter | 41 |
Lower maintenance costs | 41 |
Better air quality/controlled ventilation in the property | 39 |
Cool and comfortable indoor environment in summer | 38 |
Having a home made from sustainable materials | 38 |
The technology/features in the property | 24 |
Increasing value of the property | 18 |
Being one of the first (being a pioneer) |
11 |
No benefits in particular | 7 |
7. Technical analysis of the case study design, construction and performance
Low carbon and low energy designs:
- The case studies demonstrated a variety of designs aiming to deliver low carbon and low energy homes. Each case study design would be expected to approach or exceed the Future Homes Standard. All of the case studies used electricity for space heating and domestic hot water, deploying different types of heat pump
- Two of the case study schemes had complicated designs due to a focus on innovative solutions and a target of achieving zero carbon or zero energy bills on site. This resulted in shortcomings for both installation and use. The other 2 schemes had lower levels of innovation and complexity and had better cost and performance outcomes. This demonstrates the importance of getting the design right to increase the supply of low carbon homes in practice
- Aided by learning from their case study schemes and this project, both developers who had more complex designs are adopting simpler low carbon solutions for future projects
Performance of case study homes:
The performance of the case study homes was better than standard new homes. The performance would likely have been improved if closer attention were given to the specification and detailing of the fabric components and integration of the services.
- In 3 of the case studies, MMC were adopted for the external walls, and 2 of the schemes also used MMC for the roof construction. In some cases, the homes did not meet their airtightness targets, often due to insufficient detailing at floor edges (between MMC panels and floor slab edge), at services penetrations, and at external doors where defective door seals were identified. Thermal bridges were not always accounted for in the design and opportunities were missed to further reduce the impact of those that had been identified in the design, particularly for the development that adopted traditional construction methods;
- A common finding was the lack of integration of the services strategies within the dwelling. At one scheme, this resulted in equipment and distributed services (such as ductwork) being located in cold lofts. In other cases, continuity of insulation or the air barrier was compromised to accommodate services. This could have been avoided with better co-ordination at an early stage in the design. In case study homes, there was a wide spread of performance for space heating and hot water:
- The measured space heating energy used across the case study developments was between 2 and 5 times higher compared to that predicted by the developer’s as-built SAP. However, when the SAP input data was adjusted (to account for the observations made), the performance gap narrowed significantly. The SAP data was adjusted based on measured site values, such as air permeability or in-situ U-values. Hence, the differences tended to be due to deficiencies in the construction stage, relative to SAP assumptions. The adjusted SAP also included changes to the efficiencies of heating plant, and corrections to the geometry of the thermal envelope (for example, the developer’s SAP in one case study did not include communal elements).
- Conversely, the hot water energy use was significantly less than the developer’s SAP, and the adjusted SAP did not vary this original prediction significantly. Across case study developments, the measured hot water energy use was between 35% and 80% lower than predicted. However, many case study homes had lower occupancy than would have been assumed in SAP. It is also possible that the efficiencies of the heat pumps (used on all sites) to deliver hot water may be less in SAP than in practice: on one site a solar collector was used to pre-heat the incoming air for the heat pump, which would not have been included in the SAP model. Residents at 2 of the case study sites did advise that they had insufficient hot water and experienced longer re-heat times than they were used to. Where hot water cylinders are connected via a heat pump (with lower primary circuit temperatures), the extended reheat time should be communicated to residents to manage their expectations. The use of larger cylinders may also be preferable: in most cases a 150-litre cylinder in dwellings with more than 2 residents appeared to be insufficient to satisfy the hot water demand.
CO₂ emissions from operational energy use:
- The developers’ SAP calculations identified the schemes to be low carbon and below their respective Part L 2013 target. Where used, PV systems were very effective at helping to reduce CO₂ emissions;
- The schemes that had the greatest amount of low carbon features also had the lowest calculated CO₂ emissions. One scheme was predicted by the design team to have negative regulated CO₂ emissions (more renewable energy generated than the amount of regulated energy consumed), something that was not realised in practice. When the calculations were adjusted, the results showed higher CO₂ emissions than calculated by the developer. In one case, the adjusted emission rates were greater than the Part L target emissions using current electricity carbon emission rates;
- Despite the performance gap at each case study, the EPC rating generated by the revised SAP calculation for a sample dwelling remained the same as that derived from the developer SAP for 3 case studies, and marginally reduced for the other case study. This demonstrates the lack of granularity in the EPC rating;
- All the case study developments were 100% electric, which is in line with the Heat and Buildings Strategy. The CO₂ emissions associated with grid electricity are falling in line with the decarbonisation of the grid’s generation sources. Using the revised, lower emission rates for electricity as published in SAP 10.2, all of the schemes’ CO₂ emissions reduced to a third or less than when using SAP 2012 emissions rates.
Comfort and indoor air quality:
- In most cases, residents reported their homes were comfortably warm during the winter months, with good heat retention;
- All the case study homes had a mechanical ventilation system, and residents did not experience issues with damp, condensation or mould, which they considered to be a major benefit. Residents of 2 schemes reported that the air was dry, and this was reflected in the observed relative humidity (RH) levels where a number of homes did have levels below 40%. Below 40% RH may cause dryness for some individuals, although none of the homes were lower than 30% RH (the lowest level recommended for comfort);
- As a proxy for indoor air quality, the metabolic CO2 levels were recorded in living rooms and bedrooms. The mean levels (during occupied times) were within the threshold limit value of 1800ppm across all homes (this value is representative of the minimum ventilation rate recommended in Building Regulations Part F);
- In 2 of the case studies, the mechanical ventilation systems were set to a slightly higher level than would be necessary for satisfactory indoor air quality. Hence, this degree of over-ventilation may be a contributing factor for the air being dry in some case, and for CO₂ levels being within threshold values across the homes (the latter being unusual);
- While providing satisfactory levels of indoor air quality is important for the health and well-being of residents, there needs to be a balance associated with the energy impact of ventilation. In general, the commissioning of the mechanical systems was sub-optimal: at one site, the ventilation rates had been commissioned to a particularly high level as the ventilation systems were originally intended to deliver warm air heating. This strategy was ultimately abandoned, but the ventilation rates were not adjusted downward until residents complained about ‘cold draughts’;
- Some of the homes that were monitored during the summer months experienced temperatures above the threshold comfort temperature in bedrooms and living rooms for significant periods. Homes that offered a greater degree of cross-ventilation, and mid-floor flats, experienced lower, more manageable temperatures. For properties that experienced the highest sustained temperatures, this was due, in some cases, to a lack of openable windows in bedrooms. Some residents attempted to mitigate solar gain by fitting solar film to their windows or retrofitting external awnings. Some residents also said that they would consider air conditioning, although doing so would negate some of the carbon benefits of these homes. Two of the developers stated that they are considering including active cooling provision in future schemes – using heat pumps for both heating and cooling. Overall, this finding would suggest that overheating was not sufficiently considered in the design, where active or passive mitigation measures could have been included.
Construction costs:
- The outcomes regarding capital costs were contrary to industry perceptions shared in interviews. There were a range of additional capital costs in the case studies, from 1% to 18% extra. Yet this extra construction cost does not need to be a barrier to viability if the design proposals are efficient. The complexity of building services was proportional to additional construction cost, and so for the 2 case studies that promoted a fabric first approach with simple services, the additional capital cost was only 1% to 2.5% of the construction cost. These developers were mindful of cost and adjusted the low carbon specification to ensure the schemes remained viable.
Running costs:
- Running costs were above expectations as there was a significant performance gap in each case study. Across the case studies, the energy bills were not as low as expected at the design stage. Some residents experienced bills that were significantly higher than they had expected. This was compounded by each case study using only electricity which is more expensive than gas, and some homes did not make best use of low carbon heating and hot water systems and controls;
- Another key contributor to these higher running costs was the significant difference in unit costs of gas vs electricity, which was not mitigated by the improved efficiency of space heating and hot water generation. The failure to access time-of-use tariffs and/or benefit from export income was another contributing factor for some of the case studies;
- The case studies demonstrate that the operational cost and performance outcomes of low carbon developments are not solely dependent on the physical design and the equipment installed, but are increasingly dependent on how energy is supplied and how the homes operate as part of the broader energy system.
Skills, competencies and collaboration:
- There is a shortage of knowledge and skills relating to delivering low carbon homes (building fabric detailing and airtightness, technologies, integration, commissioning etc), which presents a challenge to scaling up the production of low carbon housing. This includes both a shortage of skilled labour on-site and a lack of capacity in the whole supply chain;
- The case studies and the wider professional interviews demonstrated a greater need for skills and experience among the teams involved in the design, as well as those building new low carbon homes;
- The more successful case studies show the importance of better knowledge and understanding of dwelling design that achieves low carbon, low running costs in practice, and dwellings that are simpler to construct and operate.
7.1 Low carbon and low energy designs
7.1.1 Case study designs
The 4 case studies demonstrate a variety of different housing designs to deliver low carbon and low energy homes. Each of the designs would be expected to approach or exceed the Future Homes Standard. While they all took a different approach, all case studies used electricity for space heating and domestic hot water, with different types of heat pump. Active Homes had a heat pump for the domestic hot water (DHW), with passive solar pre-heat, and a warm air system using electricity for the space heating, combined with the ventilation system. This warm air system was later changed to direct electric panel heaters. The successful use of electric space heating relies on a very low space heating demand, and in this case, there was on-site electricity generation via building integrated PV and battery storage. The design intention was that electricity generated on-site together with off-peak grid electricity would charge the battery. Contrary to the initial proposal, residents were not able to access the time-of-use tariffs to support charging of the battery at a lower rate tariff, as the supply was changed to 3-phase and no smart meters were available for such supplies. While the intention was to change the provider to enable access to time-of-use tariffs, this has been hindered by 2021/2022 industry-wide challenges, with customers generally being advised not to switch providers as their bills would go up dramatically. Pobl is trying to address this by switching all tenants gradually to Octopus/Tesla[footnote 30], which enables the use of the Tesla app and opens up the possibility of lowering tariffs according to time of use.
The heat pump systems in the case studies, as designed, were around 3 to 4-and-a-half times more energy efficient in the provision of space heating than a gas boiler (based on their Seasonal Coefficient of Performance) and thus they significantly reduce energy consumption in new homes. When this increased efficiency is combined with a lower carbon factor (a unit of grid-based electricity generates 35% less carbon emissions than a unit of gas[footnote 31]), a heat pump should result in less than one sixth of the emissions of a gas boiler, with savings increasing as the grid decarbonises. The electricity required by a heat pump can also be generated on site, for example via solar PV panels, with an assumed zero carbon factor.
While heat pumps were used on these schemes, there are other low carbon heat solutions which are expected to be used for some low carbon homes. These options include converting or replacing the gas network to run on green hydrogen, low carbon heat networks in areas of high-density heat demand (which may also include heat pumps), or highly insulated homes with minimal space heating provision required such as those in line with the Passivhaus standard.
In addition, for each of the schemes there were other measures to improve energy and carbon performance. This included triple glazing at 2 case studies, MVHR systems at 3 case studies (the other scheme had MEV), and solar PV at 3 case studies.
However, delivery issues in practice meant that as-designed energy and carbon targets were not achieved in practice. In particular, 2 case studies had very complicated designs due to a focus on innovative solutions, and this resulted in shortcomings for both installation and use. It demonstrates the importance of getting the design right to increase the supply of low carbon homes in practice. It also shows the importance of improving knowledge and understanding of how to design a building such that it is simpler to construct and operate to achieve the enhanced standards in practice. The developers behind the 2 case studies with complex designs have adopted simpler solutions for future projects.
7.1.2 Innovative designs and use of SAP
Some innovative case study features could not be applied in SAP. Attendees at the energy systems workshop held as part of the project raised SAP as a barrier to innovation in some cases. While SAP is a compliance tool, not a design tool, designs often focus on what is more cost-effective to achieve compliance via SAP. Conversely, if the benefits of a technology or combination of technologies are not reflected in SAP, this acts as a deterrent to its adoption. SAP therefore has a large impact on the design strategy selected. However, it is important to ensure the SAP methodology is robust, and any benefits from innovative technologies are evidence-based, which impacts on the timeline of including new technologies in SAP and how they are represented.
In 3 of the case studies, the innovative scheme features were not fully accounted for in SAP 2012 (Active Homes, Etopia Homes and Tallack Road). All 3 had issues around specific innovative features. For Active Homes and Etopia Homes there was an inability to model PV batteries in SAP 2012. At Tallack Road, where an approved methodology was agreed for the ambient loop communal ASHP system with in-dwelling water-to-water heat pumps to be included in SAP, it was unclear whether this accurately represented the system (heat pump efficiencies from in-dwelling heat pumps, distribution losses, thermal storage including impact on efficiency).
Two of the case studies also had issues with representing the integration of low carbon features. For Active Homes, there was a challenge in allowing for the impact of TSCs on ASHP efficiency. For Etopia Homes, SAP 2012 did not allow for solar thermal energy from the Photovoltaic-thermal (PVT) panels to offset space heating demand and SAP did not allow for the impact of EEB on GSHP efficiency. Issues relating to more complex interaction between technologies are ongoing.
The potential operational cost and carbon benefits from incorporating energy storage on-site was raised. This could be through thermal or battery storage, including the use of electric vehicles. Storage allows residents to maximise the use of energy generated on-site. In addition, time-of-use tariffs allows them to import energy from the grid during periods of lower cost tariffs, when the grid is also generally lower carbon, and to use stored energy during periods of higher tariffs when the grid is generally higher carbon. SAP would need to be more dynamic to capture such benefits.
If the innovative features described above operate as intended, further related carbon emissions reductions or energy cost reductions may be anticipated (beyond those captured in SAP).
7.2 Performance of case study homes
7.2.1 Fabric
The fabric U-value performance was generally better than the standard new home (Part L 2013) but most homes missed their airtightness targets and significant issues were identified with thermal bridging (including bridges not being accounted for in the design).
Particularly for a low carbon home with electric heating, there is a closer coupling between the fabric and the heating system. Better fabric performance will help to lower the heat demand, which in turn will allow heat pumps to operate more efficiently and at lower flow temperatures. Even allowing for high efficiencies of heat pumps, as highlighted in Section 7.5.2, the cost per unit of fuel is higher for electricity than gas, so reducing the heat demand through improved fabric efficiency will be a further benefit for lowering energy bills. Therefore, the detailing of the building fabric requires more attention at both design and construction stages to fully realise the benefits. The importance of the detailing of junctions, interfaces, and penetrations needs to be clearly conveyed to site staff, particularly between different trades: a point that appears not to have been fully understood to date. One case study used the Passivhaus methodology (PHPP) at early stages, and 2 others referred to a ‘better than Passive’ approach (either ‘Active’ or ‘Energy plus’), but none of the case studies continued the Passivhaus approach after planning stages. This meant that some of the benefits of the standard, including greater attention to construction detailing and third party on-site quality audits, were not realised.
There were some common areas of underperformance: a sample of which are illustrated in the thermal images below.
Figure 14: Thermal image (and accompanying visual) indicating thermal bridge associated with structural steel within soffit and connected column
Figure 15: Thermal image (and accompanying visual) indicating air leakage on external door lower seal and at threshold
Figure 16: Thermal image (and accompanying visual) indicating air leakage at external wall junction where compressive seals between MMC wall panel and floor are compromised
7.2.2 MMC
Three of the 4 case studies made use of panelised solutions (MMC), either a timber or steel SIP or a CLT system. In each case, site observations and post-completion testing demonstrated that the panelised solutions had the capability to deliver higher thermal performance than traditional construction, particularly with reduced thermal bridges. However, the levels of airtightness achieved were worse than intended.
The poorer air permeability largely related to a lack of suitable planning and coordination of components. This resulted in system-build components being badly interfaced - or incompatible - with site-build junctions, rather than leakage between MMC element junctions, such as:
- compromised edge seals at Marmalade Lane or incompatible groundworks at Etopia Homes
- traditional cold roof at Active Homes, which created potential thermal bypass zones
- building services penetrations through the panelised systems
As observed in the case studies, such issues can also slow the pace on site and reduce or compromise the potential performance and speed benefits of MMC. The development teams, however, said they have learned from these projects. In some cases, the case study development was their first experience of such MMC systems, and they expect to make improvements to delivery in the future.
7.2.3 Design integration and continuity
A lack of design integration led to performance gaps. Examples from the site observations included services clashing with insulation and impacting on the airtightness of the homes. With their complex design and installation programmes, Etopia Homes and Active Homes suffered from poor on-site co-ordination and interfacing with integrated systems. Construction quality was poorest in the schemes with complex M&E, and a lack of co-ordination between trades was observed. Furthermore, essential technologies and plant was sometimes relegated to ill-thought-out locations, for example, the MVHR being installed in cold lofts at Active Homes compromised performance and increased space heating losses.
Figure 17: Example of MVHR and heater unit in cold loft with sections of uninsulated ductwork
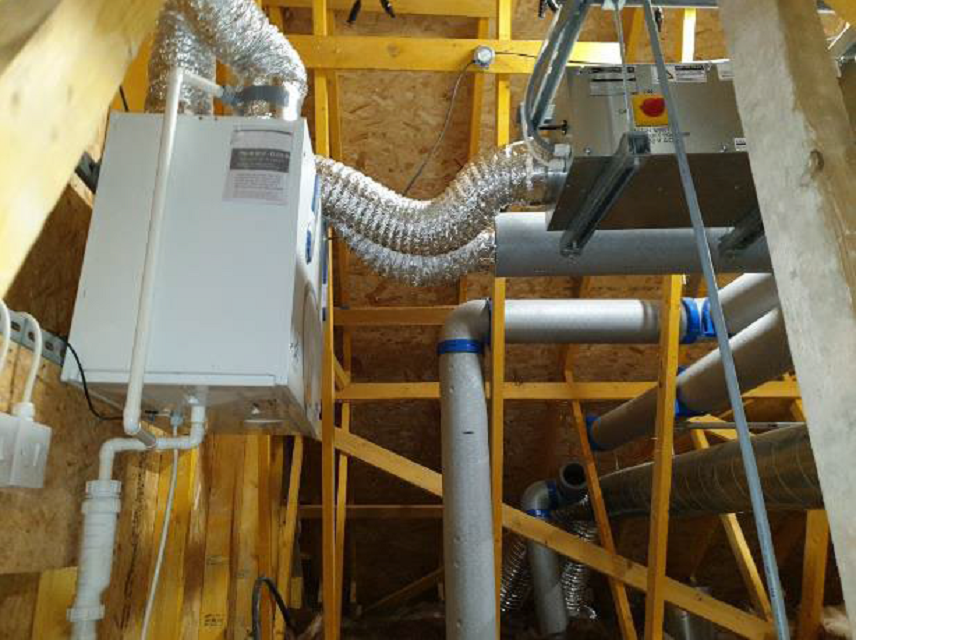
There were also significant design changes without the involvement of the original design team and an understanding of the impact which had significant consequences, for example:
- Active Homes: An initial fabric standard was selected that matched the capacity of the heating system solution. However, the fabric standard was reduced during subsequent stages and upon completion the heating system did not have sufficient capacity to heat the homes during winter. Considerable time and expense were necessary to understand and resolve the issue, which resulted in the installation of a new heating system. Furthermore, the solution is expected to result in high on-going bills for residents (see 7.5.2);
- Etopia Homes: A key feature of the design was the use of the GSHP for space-heating, pre-heated by the Earth Energy Bank (EEB), itself supplied by PV-Thermal panels (PV-T) on roofs. The EEB was located below houses to provide inter-seasonal storage. When the developer changed, the ground floor and foundation designs were revised which unintentionally impacted on the performance of the EEB. There were also some installation issues. Taken together, this is expected to have significantly reduced the GSHP efficiency, which was reportedly often operating in electric boiler mode (meaning that at a CoP of 1, the design Seasonal Coefficient of Performance (SCoP) was just over 4.5). This arose partly because of the complexity of the system and the lack of engagement with the original design team.
7.2.4 Space and water heating
The intention was to monitor the energy consumption and environmental conditions for at least 12 months on each site. However, this was not always possible due to construction delays, the associated delays to residents moving in, and restricted access to homes during the COVID-19 pandemic to install equipment. The research programme was extended to accommodate these delays, but in most cases the monitoring stage was shortened. The period of monitoring is shown in Table 1.
Table 1: Monitoring period for each case study
Case study | Energy data | Environmental data |
---|---|---|
Marmalade Lane (ML) | Data collected for 18 months - February 2019 to August 2020. Months 6 to 18 (a 12-month period) used for final analysis.* |
Data collected for 18 months - February 2019 to August 2020. Months 6 to 18 (a 12-month period) used for final analysis.* |
Active Homes (AH) | 3-to-4-month period (depending on the home) between December 2020 and March 2021 | 3-to-4-month period (depending on the home) between December 2020 and March 2021 |
Etopia Homes (EH) | No data | 8-month period from August 2020 to March 2021 |
Tallack Road (TR) | 7 months from September 2020 to March 2021 | 4-to-7-month period (depending on the home) between September 2020 and March 2021 |
*Although Marmalade Lane was monitored for 2 summer periods, the research team selected the second summer for the following analysis as this overlapped with the summer data for Etopia Homes. Interestingly, despite July 2019 having the hottest day on record, summer 2020 was warmer, on average.
The research team has determined the space heating and hot water consumption, and compared to SAP as a benchmark, for 3 schemes (ML, AH, TR)[footnote 32]. Energy data was unavailable for the Etopia Homes site. Instead, a comparison has been made between developer SAPs and Building for 2050 (Bf2050) SAPs: the latter being adjusted to reflect observed performance issues.
The space heating and hot water energy use for the 3 monitored schemes is provided in Table 2. This table is supplemented by the graphs in Figure 18 and Figure 19, which summarise the space heating and hot water energy use across the case study developments. The different monitoring periods have meant that a direct comparison of the space heating and hot water fuel use across the developments is not possible. Instead, the energy data has been converted to a ratio against the SAP 2012 Part L limit, where:
- the median actual fuel use is given, separated by houses and flats (where both are present);
- the median SAP-predicted energy consumption for the monitored homes, separated by houses and flats (where both are present). This is shown as the ‘Developer’s Design’ and is based on the developer’s as-built SAP;
- the research team (Bf2050) SAP for a representative dwelling[footnote 33] is included as ‘Adjusted Design’. This is based on the developers’ SAP models, which have been adjusted to include for site observations and post-completion testing by the research team.
To improve the comparison against the monitored data, adjustments have been made to the calculated SAP space heating energy to account for the mean external monthly temperature data recorded from a weather station installed on each case study development as part of the monitoring. This significantly changes some of the SAP energy consumption predictions.
Table 2: Measured space heating and hot water energy use for each case study
Case study | Type | Space Heating Energy (total kWh during monitored period) Median (kWh) |
Space Heating Energy (total kWh during monitored period) Range (kWh) |
Domestic Hot Water Energy (total kWh during monitored period) Median (kWh) |
Domestic Hot Water Energy (total kWh during monitored period) Range (kWh) |
---|---|---|---|---|---|
Marmalade Lane (ML) | Houses | 1,875 | 1,528 to 2,183 | 392 | 245 to 1,063 |
Marmalade Lane (ML) | Flats | 1,296 | 914 to 1,965 | 260 | 134 to 473 |
Active Homes (AH) | Houses | 2,603 | 1,192 to 3,403 | 218 | 186 to 390 |
Active Homes (AH) | Flats | 1,284 | 877 to 4,609 | 217 | 166 to 355 |
Tallack Road (TR) | Flats | 884 | 289 to 1,668 | 180 | 112 to 369 |
The results demonstrate a wide spread of performance for space heating, which was higher compared to that predicted by the developer’s as-built SAP. Conversely, for hot water, the energy use was less when compared to the developer’s SAP. The reasons for this are further explored in Table 3 and Table 4.
Figure 18: Space heating fuel use across the case study developments
Description of Figure 18:
Chart showing the space heating fuel use across the 4 case study developments. It shows that the performance for space heating was higher compared to that predicted both by the developer’s original design and their adjusted design. The actual fuel use in the Active Homes flats in particular was significantly higher than predicted.
More detail is provided in tables 2, 3 and 4.
Figure 19: Domestic hot water fuel use across the case study developments
Description of Figure 19:
Chart showing the domestic hot water fuel use across the across the 4 case study developments. It shows that the the energy use was for hot water was less than predicted by both by the developer’s original design and their adjusted design.
More detail is provided in tables 2, 3 and 4.
Table 3 and Table 4 summarise the space heating and domestic hot water end uses, respectively. They highlight the differences between the actual (measured) energy against the developer’s as-built SAP, and the research team adjusted SAP calculations. The reasons for the differences are discussed alongside.
It should also be noted that SAP is not an accurate prediction of actual energy use, relying on standard assumptions around occupancy, heating patterns and hot water consumption, for example. The weather-adjustment of the SAP data for the space heating will compensate for these differences to some degree, but the standard assumptions in SAP will still limit any comparisons and may partly explain any differences observed between SAP calculations and measured data. In all case studies, residents’ behaviour will have had a large impact on the energy use, which relates to their preferences and practices, their lack of familiarity with the installed systems and controls, and – in the view of some residents – the lack of effective guidance provided by the developer. Such issues are discussed further below.
All schemes were assessed during the COVID-19 pandemic, including lockdown periods when more residents worked from home and/or stayed at home, which will differ somewhat from the standard occupancy assumptions included in SAP. Furthermore, all monitored results are based on the first year of occupancy (except for Marmalade Lane which includes a twelve-month period, covering both the first and second year of occupancy) and it may be expected that energy-using behaviour may change as residents become more familiar with their heating system.
Table 3: Space heating energy use compared to design
Marmalade Lane
How actual performance compared to the design assumptions (based on SAP):
- The median annual space heating energy measured for both the houses and flats was around 3 times higher than that predicted by the developer as-built SAP;
- The research team’s as-built SAP results, which were adjusted to include site observations and post-completion testing, better reflect measured space heating energy use. There is a still a significant gap for the houses;
- The median annual space heating energy measured for both the houses and flats was around 30-35% below the Part L 2013 standard (using the notional building as a comparator).
Potential reasons for differences:
There were several reasons identified for why the measured space heating energy use was higher than that predicted by the developer’s SAP calculations:
- In the houses, the fabric performance was poorer than in the developer’s SAP in some cases. For example, there was air leakage at floor edges where prefabricated panels met in-situ components, with external door seals, services penetrations through ceilings and boxings, and with incoming services. The research team’s tested air permeability was more than twice that used in the developer’s SAP. Roof insulation in the houses was less than specified due to clashes with services and truss design, and some thermal bridging issues were identified that were not accurately reflected in the SAP calculations;
- The communal system serving the flats included heat metering. Investigations showed the efficiency of the heat pump (seasonal co-efficient of performance) was around half that used in the developer’s SAP and around 50% of this difference was due to distribution heat losses;
- The MVHR system air flow rates measured were out of balance when operating at background ventilation setting. These systems should be commissioned such that the intake and exhaust air flows are balanced, thereby maximising the amount of air passing via the heat exchanger. In this case study, the exhausted air flow was higher than the intake, which would increase the ventilation heat losses from the properties and lower the efficiencies of the heat exchangers.
It is unclear why the research team’s SAP is not closer to the actual energy consumption for the houses. Measuring the performance of the individual low carbon technologies was outside of the scope of this study. There was no heat metering of the individual ASHPs serving the houses and their performance may also be less than assumed in SAP. Manufacturer’s data for the heat pump efficiencies was used.
The finding that the measured energy use is significantly below the Part L 2013 target is driven by the use of a heat pump. The Part L specification assumes a gas boiler with 93.5% efficiency. By contrast, the efficiencies for the heat pumps used are expected to be better than this (assumed in the developer and research team’s SAP calculations to be between one-and-a-half to 4 times more efficient than a gas boiler).
Active Homes
How actual performance compared to the design assumptions (based on SAP):
The measurements were limited to a 3-to-4-month period, subject to residents’ moving in dates.
- The median space heating energy use measured in the houses was more than 3 times higher than that predicted by the developer as-built SAP. The measured energy use in the flats was around twice that predicted by SAP;
- The research team’s as-built SAP results, which were adjusted to include for site observations and post-completion testing, better reflect measured space heating energy use, albeit there is still a significant gap for the houses;
- The median annual space heating energy measured for the houses was around 75% above the Part L 2013 standard (using the notional building as a comparator). This was improved for the flats, but still 10% higher than the Part L 2013 standard.
Potential reasons for differences:
There were several reasons identified for why the measured space heating energy use was higher than that predicted by the developer’s SAP calculations:
- Significant fabric performance issues were identified, such as poorly applied roof insulation and, although not confirmed, significant thermal bypass is expected due to service zones between the internal plasterboard lining and SIPs construction into the cold loft;
- The top floor flats, which had the greatest performance gap, included an access stairwell within the thermal envelope leading to a hallway on the first floor. The stairwells (which are not provided with any heating equipment) account for approximately 15% of the total volume and have not been fully accounted for in the developer’s SAP calculations;
- The MVHR system air flow rates measured were out of balance when operating at background and boost ventilation settings. These systems should be commissioned such that the intake and exhaust air flows are balanced, thereby maximising the amount of air passing via the heat exchanger for optimum efficiency. In this case study, the systems were significantly out of balance with air flow priority given to the supply air. This imbalance is thought to be due to the original warm-air heating strategy (subsequently abandoned) where the heating element on the supply air needed greater air flow to operate the heater safely[footnote 34]. It was also found that the MVHR equipment and the majority of distribution ductwork were located in the cold lofts. Locating these services outside of the thermal envelope would result in greater distribution heat losses and would further reduce the efficiency of the heat exchangers. Some residents did complain about ‘cold air’ coming from the supply air diffusers.
It is unclear why the research team’s SAP is not closer to the actual energy consumption for the houses. It was noted that SAP does not account for the thermal bypass identified above which is a potential cause of this difference.
The finding that the measured energy use is above the Part L 2013 target reflects 2 issues. The first is that the Part L 2013 target specification assumes a gas boiler with 93.5% efficiency, which is similar to the efficiency that the electric panel heater is likely to achieve. As above, fabric issues have been identified which reduce the energy performance of the building below that assumed for the Part L 2013 target.
Etopia Homes
How actual performance compared to the design assumptions (based on SAP) with reference to dwellings with GSHPs[footnote 35]
It was not possible to measure the energy use on this development, and the developer was unable to provide in-use data for analysis. Comparisons with SAP data have instead been made.
- The annual space heating energy use was around 2.5 times higher for the research team’s as-built SAP compared to the developer’s as-built SAP on a representative property;
- The predicted annual space heating use from the developer’s SAP was a factor of 6 below the Part L 2013 standard (using the notional building as a comparator).
Potential reasons for differences
A key reason for the difference between the developer and research team’s SAP results were additional fabric heat losses identified by the research team. In particular, the research team’s air permeability results (2.4 m³/h.m² @ 50Pa) were significantly higher than those of the developer (0.3 m³/h.m² @ 50Pa). While the research team’s test result is still much better than that needed for Building Regulations compliance, it is, nonetheless, 8 times higher than the result obtained by the developer for use in their SAP calculations. This may be due to poor fitting window and door seals observed (see Figure 15), and issues with sealing incoming services. Another key reason for the difference in SAP results was reported issues which reduced the GSHP efficiency. This may be due to errors with the installation of earth energy bank sensors and the use of a sub-optimal foundation design which increased water flow and heat transmission through the earth energy bank.
The predicted energy use was significantly below the Part L standard as the building uses heat pumps, whereas the Part L standard is based on a significantly less efficient gas boiler.
Tallack Road
How actual performance compared to the design assumptions (based on SAP):
The measurements were limited to a 6-month period.
- The median annual space heating energy measured for the flats was around 4 to 5 times higher than that predicted by the developer’s as-built SAP;
- The research team’s as-built SAP results, which were adjusted to include site observations and post-completion testing, better reflect measured space heating energy use, albeit there is still a significant gap remaining.
The median annual space heating energy measured for the flats was around 10% below the Part L 2013 standard (using the notional building as a comparator).
Potential reasons for differences:
There were several reasons identified for why the measured space heating energy use was higher than that predicted by the developer’s SAP calculations:
- There were additional fabric heat losses to those assumed in the developer’s SAP. This includes poorer thermal performance of the uninsulated communal walls between the flats and unheated corridors, more thermal bridging than identified in the design, and exposed upper floors, soffits and undercroft areas;
- The efficiency of the heat pumps may differ from that assumed in SAP. The ambient loop heat pump approach has been granted an approved methodology for inclusion in SAP[footnote 36]. However, it is questionable whether this accurately represents the system. The presence of a medium temperature thermal store and an extended external pipe run may also affect system efficiency.
The research team’s SAP is much closer to the measured energy use. As noted above, the actual efficiencies of the ambient loop heat pump system may at least partly explain the remaining difference.
The finding that the measured energy use is 10% below the Part L 2013 target is driven by 2 balancing factors. The Part L specification assumes a gas boiler with 93.5% efficiency. By contrast, the efficiencies for the heat pumps used are expected to be better than this. However, the fabric and services issues referenced above largely offset this and bring the data into alignment.
Table 4: Domestic hot water energy use compared to design
Marmalade Lane
How actual performance compared to the design assumptions (based on SAP):
- The measured median annual hot water energy was significantly lower than that predicted by the developer as-built SAP – around 80% lower for houses and 60% lower for the flats;
- The research team’s as-built SAP results were similar to those of the developer;
- The median annual hot water energy measured for both the houses and flats was around 90% below the Part L 2013 standard (using the notional building as a comparator).
Potential reasons for differences:
There were several reasons identified for why the measured hot water energy use was lower than that predicted by the developer’s SAP calculations:
- Most homes have lower occupancy than the SAP assumption. In some cases, 3- or 4-bedroom homes may be occupied only by 2 or 3 people. The SAP prediction of hot water consumption is significantly dependant on occupancy;
- Residents of this case study engaged with each other to identify ways to reduce energy use;
- It is possible that the efficiencies of the ASHP to deliver hot water may also be less in SAP than in practice. Heat pump efficiencies were based on manufacturer’s data.
As for space heating, the finding that the measured energy use is significantly below the Part L 2013 target is driven by using a heat pump, whereas the Part L specification assumes a significantly less efficient gas boiler.
Active Homes
How actual performance compared to the design assumptions (based on SAP):
The measurements were limited to a 3-to-4-month period, subject to residents’ moving in dates.
- The measured median hot water energy use was around 40% to 50% less than predicted in the developer’s as-built SAP calculations;
- The research team’s as-built SAP results were similar to those of the developer;
- The median annual hot water energy measured for both the houses and flats was around 60% below the Part L 2013 standard (using the notional building as a comparator).
Potential reasons for differences:
There were several reasons identified for why the measured hot water energy use was lower than that predicted by the developer’s SAP calculations.
- The effect of the TSCs (not included in SAP) is expected to be a contributing factor for the lower hot water energy observed. This should increase the efficiency of the hot water system by pre-heating the air supplied into the heat pump cylinder. The specified ASHP cylinder was not available in the SAP database and so the default was used in SAP, which also contributes to the difference;
- Some residents advised that they had insufficient hot water. Residents had relatively small 150 litre cylinders (which should be sufficient for the flats, but possibly too small for 3- or 4-bedroom houses), and relatively long re-heat times (55°C hot water circuit for the heat pump increases time to re-heat the cylinder). Residents may have previously had a combination system (which provides instantaneous hot water) or a standard boiler with a larger or quicker re-heating hot water cylinder. The slower recharge rate may have reduced hot water use, and this may increase in time as residents become more familiar with the system.
The finding that the measured energy use is significantly below the Part L 2013 standard is driven by using a heat pump for hot water, the use of the TSC, and residents’ use of hot water.
Etopia Homes
How actual performance compared to the design assumptions (based on SAP):
Energy use was not measured on this development as the intention was for the developer to provide this data.
- The annual hot water energy use estimated by the research team’s as-built SAP was similar to the developer’s as-built SAP on a representative property;
- The predicted annual hot water energy use from the developer’s SAP was a factor of 4 below the Part L 2013 standard (using the notional building as a comparator).
Potential reasons for differences:
The finding that the predicted energy use is significantly below the Part L 2013 standard is likely driven by using a heat pump for hot water, which has significantly improved efficiency compared to the gas boiler used for the Part L specifications. This difference is further amplified by the presence of wastewater heat recovery, which reduces the demand for hot water relative to that in the Part L 2013 specification.
Tallack Road
How actual performance compared to the design assumptions (based on SAP):
The measurements were limited to a 6-month period.
- The measured median hot water energy use was around 35% less than predicted in the developer’s as-built SAP calculations;
- The research team’s as-built SAP results were similar to those of the developer. The measured median hot water energy use was around 80% below the Part L 2013 standard (using the notional building as a comparator).
Potential reasons for differences:
One reason was identified for why the measured hot water energy use was lower than that predicted by the developer’s SAP calculations - some residents said that they had insufficient hot water (150 litre cylinders installed), and longer re-heat times than they were used to (55°C re-heat circuit). It is possible that hot water energy may increase in time.
The finding that the measured energy use is significantly below the Part L 2013 standard is likely driven by using a heat pump for hot water which has significantly improved efficiency compared to the gas boiler used for the Part L specifications.
7.3 Carbon emissions from operational energy use
SAP analysis suggests that the scheme designs are generally low carbon, particularly when decarbonisation of the electricity grid is considered, although operational issues can significantly affect actual carbon emissions. Figure 20 shows the carbon emissions for representative units based on SAP calculations compared to the Part L 2013 standards[footnote 37]. SAP 2012 has been used for this analysis as this is the version of SAP used for demonstrating compliance with Part L 2013. However, the carbon emissions in this version do not reflect the current level of electricity grid decarbonisation (this is addressed in later versions of SAP for use with Part L 2021). Most of the case study schemes feature innovative technologies, which are not well accounted for in SAP 2012. This means that anticipated CO₂ emission reductions may not be accurately reflected.
- Two Part L standards are included in the chart. The target CO₂ emissions for electrically-heated homes (applicable to the case study schemes), and the equivalent target for gas-fuelled homes (to represent standard new build housing). The Part L 2013 target for electrically heated homes is relaxed relative to the target for gas[footnote 38]. It is recommended that the target for gas is used for the comparison here as it is the core Part L target;
- Both the developer and Bf2050 research team SAP-calculated CO2 emissions are shown. These reflect the differences between normal compliance-based assumptions (developer SAP) and where adjustments have been made by the research team arising from site observations and post-completion fabric testing. This highlights key performance gap impacts;
- The Bf2050 SAPs adjusted to SAP 10.2 are also shown, to demonstrate the performance of the schemes assuming the Part L 2021 carbon emission factors apply, which reflect a more decarbonised electricity grid representative of 2020-2025.
Figure 20: Predicted CO₂ emissions across the 4 case study developments
Description of Figure 20:
Bar chart showing the predicted carbon emissions across representative units in the 4 case study developments, based on SAP calculations, compared to the Part L 2013 standards. Details below.
The following points were noted:
- all of the developer SAP CO₂ emissions are below the Part L 2013 gas target, except for the Marmalade Lane houses which are marginally above the target. Note that the Marmalade Lane houses are compliant with Part L, as they need to meet the target for electricity. Marmalade Lane is the only scheme without solar PV installed, which is effective at reducing CO₂ emissions for the other schemes;
- Active Homes and Etopia Homes are the schemes with more low carbon features, and they were predicted to have the lowest CO₂ emissions. The developer predicted Active Homes to have negative regulated carbon emissions, meaning more renewable energy generated than the amount of regulated[footnote 39] energy consumed;
- the Bf2050-adjusted SAPs all differ from the developer SAPs, reflecting the performance gap shown in the previous section, and consequently show significantly higher CO₂ emissions. The Marmalade Lane flats and Active Homes dwellings (houses and flats) show the largest gap between developer SAPs and Bf2050 adjusted SAPs, and Etopia Homes has the smallest difference. A key reason for the Etopia Homes results was that the research team observed, and accounted for, nearly twice as much solar PV than included in the developer’s SAP (this was believed to be an error);
- the Bf2050-adjusted SAP CO₂ emissions are above the Part L 2013 electricity target for the Marmalade Lane flats, and similar to the Part L 2013 gas target for both the Marmalade Lane houses and Tallack Road. However, as highlighted in Table 3, the actual energy performance was often significantly poorer than the Bf2050-adjusted SAPs;
- all of the case study schemes run entirely on grid electricity, which has rapidly declining associated CO₂ emissions. Generally, these schemes show significantly lower carbon when CO₂ emissions factors that reflect current decarbonisation (meaning factors from SAP 10.2) are used. Over the next decades, CO₂ emissions from such all-electric schemes are expected to reduce further as the grid continues to decarbonise.
As noted above, the research team created a Bf2050-adjusted SAP, and this was compared with developer SAP. In each case, these generated an Energy Performance Certificate (EPC) rating, band A representing the best performance. Notably, the EPC for the Bf2050-selected sample dwelling remained the same as that derived from the developer SAP in each case, except for one which marginally reduced. This demonstrates the lack of granularity in the EPC rating, see Table 5 below.
Table 5: Comparison of EPC ratings
EPC rating/Case study | EPC from developer SAP calculation | EPC from Bf2050 SAP calculation |
---|---|---|
Marmalade Lane | B (83) | C (78) |
Active Homes | A (103) | A (92) |
Etopia Homes | A (99) | A (97) |
Tallack Road | B (85) | B (81) |
Assessing embodied carbon emissions in case study scheme was beyond the scope of the research. If these were calculated retrospectively, assuming the required data could be obtained, then a whole lifecycle carbon assessment could be determined, drawing on the embodied and operational carbon emissions.
7.4 Comfort and indoor air quality
Residents expected good thermal comfort to be a benefit of living in low carbon homes. This was realised in practice, with residents reporting their homes being warm during the winter months with good heat retention. This was confirmed by our monitoring of homes in all 4 case studies, which showed that the heating systems had sufficient capacity and delivered comfortable temperatures (>18°C). An exception to this was Active Homes where some residents did have issues in heating their homes, at least partly due to the overly complex control system and insufficient training on how to use it, but also reducing space heating use due to the cost of heating using direct electric panel heaters.
The monitored temperature ranges in each home across the developments are summarised in Figure 21. The median temperatures were above the 18°C threshold, with the main exception of some homes at Active Homes which had significant periods of temperature below this (for the reasons given above). In many homes, some periods were below 18°C and this was usually during the night-time, when either the heating was programmed to be off, or during short periods of non-occupancy (such as holidays). Some residents also selected lower setpoint temperatures due to either comfort preference or wishing to reduce energy bills, or possibly to reduce environmental impacts.
Figure 21: Mean internal temperature in winter with median and quartile ranges
(_H denotes houses; _F denotes flats)
Description of Figure 21:
Chart showing the monitored temperature ranges in each home across the developments. Details above.
All of the case study homes had a mechanical ventilation system. Very few residents experienced any issues with damp, condensation or mould and they considered this to be a major benefit after some previously lived in homes with such problems. This graph shows the RH conditions inside the living rooms in each monitored home. The living room is chosen to be representative in this case, as all of the homes on 3 of the developments had open plan living/dining and kitchen areas. The exception was Active Homes, where the monitored RH levels in the living room were similar to those in the kitchens. The mean RH in most case study homes was within the 40% to 60% range, although minimum values in 10 of the homes across the developments fell below 40%. However, none dropped below 30%, the lower limit recommended to prevent dryness of mucous membranes. To minimise the risk of condensation and mould on surfaces, Approved Document F recommends a RH limit of 65% using a 30-day moving mean. Applying this criterion, the 65% threshold was exceeded in only one property at Tallack Road (TR_F1), where the under-ventilation in this case was thought to be a factor.
Residents of 2 schemes reported that the air was dry, and this is supported by the research team’s monitoring. Figure 22 shows the mean relative humidity (RH) values at Marmalade Lane and Etopia Homes were lower than at the other 2 schemes, with the daily minimum values dropping below 40% in some homes[footnote 40]. Both developments used MVHR systems, and it is likely that one of the reasons for the dryness was due to higher than necessary air flow rates being commissioned. Furthermore, the information provided to residents about the operation of their systems could have been clearer (residents reported a lack of clarity of how their ventilation systems were meant to work). Residents of Active Homes reported cold draughts – probably due to mis-installing the MVHR system in a cold loft (thus resulting in greater heat loss), the positioning of ventilation diffusers in the rooms, and the higher air flow rate (the systems were initially intended to supply warm air, this was later changed to fresh air only). There was also a misunderstanding by some who thought, incorrectly, that their homes had air-conditioning installed.
Figure 22: Daily RH levels in winter in living rooms using 30-day moving mean with min/max range
(_H denotes houses; _F denotes flats)
Description of Figure 22:
Chart showing the mean relative humidity (RH) in winter in living rooms. Details above.
Figure 23 summarises the carbon dioxide (CO₂) levels observed in the main bedrooms in each monitored home. A threshold value of 1830 parts per million (ppm) has been added, as this corresponds with the steady-state CO₂ equilibrium when applying the minimum background ventilation rate (3.5 l/s) recommended in Approved Document F. The analysis for the CO₂ concentrations observed is based on the mean hourly values for assumed occupied periods in the bedrooms (22:00 to 07:00), applying a 9-hour rolling average. The mean values were lower than the threshold value in all properties, although AH_F4 was the highest mean at 1770ppm. This higher level is thought to be due to a period where the MVHR unit was performing sub-optimally because residents adjusted settings. The maximum value exceeded the threshold in 7 dwellings in total, with 5 of these homes being at Active Homes where problems with the MVHR installations were found (as discussed in Table 3). At Tallack Road, the same flat (F1) had the highest RH. This flat, along with 2 other flats in this development, had the highest maximum RH values. On this development, ventilation performance (poor mixing due to lack of air inlets) was a likely contributing factor.
Figure 23: CO₂ levels in winter in main bedrooms during occupation using 9-hour moving mean with min/max range
(_H denotes houses; _F denotes flats)
Description of Figure 23:
Chart showing the carbon dioxide (CO₂) levels observed in the main bedrooms in each monitored home. Details above.
Residents from all the case studies reported a lack of clarity about how to operate their mechanical ventilation systems (3 case studies had MVHR and one had MEV). They suggested that there should be better advice provided by developers and those handing over properties, to inform residents of the benefits of good operation of their homes. The case studies demonstrated that efficient operation may differ from what residents are used to. For example, some residents were told that they should not need to open their windows as their ventilation systems should bring in fresh air (and MVHR systems are more efficient with windows closed), but some residents have a habit of opening them and felt that they still needed to or wished to.
Two of the case studies were reviewed post-occupation over a summer period (Marmalade Lane and Etopia Homes). In both cases, there was overheating in the homes – evidenced both in reports from residents and in observations made by the research team, considering the following criteria (based on CIBSE Guide A):
bedrooms not to exceed internal temperatures of 26°C during occupation, and living rooms not to exceed 28°C during occupation.
Figure 24 and Figure 25 show the temperatures recorded in the main bedrooms and living rooms. The charts present the quartile ranges of the temperatures observed during the monitored summer months (beginning of May to end of August), and during standardised occupied times: 22:00 to 07:00 for bedrooms and 12:00 to 22:00 for living rooms. With the exception at Marmalade Lane in ML_F6, which is the only ground floor flat, and ML_F7, which is the only mid-floor flat, all bedrooms experienced temperatures above the threshold of 26°C for a significant period, with all houses experiencing higher temperatures for greater periods. It is notable at Etopia Homes that EH_H2 experienced the highest temperatures across all monitored bedrooms, despite the monitored bedrooms in this development being located on the ground floor (they had no openable windows – only French doors, which were kept closed overnight for security).
Figure 24: Summer temperature in monitored bedrooms
(_H denotes houses; _F denotes flats)
Description of Figure 24:
Chart showing the temperatures recorded in the main bedrooms. Details above.
In most cases, the temperatures in the living rooms are similar to those of the bedrooms. Given the higher threshold temperature for living rooms (28°C), it was exceeded for less time.
Figure 25: Summer temperature in monitored living rooms
(_H denotes houses; _F denotes flats)
Description of Figure 25:
Chart showing the temperatures recorded in living rooms. Details above.
Some residents were looking at additional physical measures to help mitigate overheating, such as adding solar films to their windows. Some were considering air conditioning which, if added, would at least partly negate the energy and carbon benefits of these homes, particularly if less efficient portable air conditioning were to be used (in comparison with more efficient fixed units), and some were trying portable fans. This was after trying behavioural measures that were possible within the constraints of the designs and the context of concerns such as security, for example, window opening, and keeping curtains/blinds closed.
Two of the developers reported that they are considering including active cooling provision in future schemes – using heat pumps for both heating and cooling.
7.5 Cost
7.5.1 Capital cost
All of the case studies highlighted a cost premium associated with the inclusion of low carbon measures. The exceptions were some low carbon MMC building fabric solutions which have the potential to be similar in cost to traditional construction, while delivering improved thermal performance. The low carbon solutions selected, and the associated cost, were partly influenced by the source of funding – though other factors were also relevant. Both Marmalade Lane and Tallack Road relied principally on sales for their income and focused on the adoption of simpler solutions at lower cost, with both adopting heat pumps. In addition, Tallack Road included PV, and Marmalade Lane included a panelised fabric solution and MVHR. Active Homes and Etopia Homes both had innovation funding to support their adoption of a more expensive, broader range of low carbon technologies. In both latter cases, the development teams intend to apply a simpler, lower cost, but still low carbon, solution in current and future schemes that do not have innovation funding.
Most of the increased cost compared to higher carbon schemes/more traditional new build homes was the cost of design, supply, and installation of the low carbon technologies, which is demonstrated in Table 6. Some schemes also had increased costs arising from additional design fees, lengthier programmes, or the use of specialist contractors and sub-contractors. The reviews undertaken for the case studies suggested that each of these costs could likely have been reduced through optimised design advice – for example through implementing simpler designs, and by ensuring design continuity and a better understanding of the impacts of design changes. The cost premium has already reduced for subsequent schemes being led by the same developers, where cost savings are being achieved by an expanding supply chain, leaner design solutions, and greater familiarisation with the technologies.
The additional construction cost of low carbon homes at scale is around 1% to 2.5% above that necessary to comply with Building Regulations. More details are provided in Table 6.
- The more innovative designs had additional costs of around 9% to 18%, with the innovation partly or fully funded by a grant.
- The non-grant funded schemes incurred additional costs of around 1% to 2.5%. This additional construction cost was absorbed into the viability model by accepting a reduction in profit rather than adding to the sales price, with a view to applying the learning and optimising designs at future schemes. At Marmalade Lane, one of the joint venture developer partners was bringing an MMC product to the UK market.
Table 6: Extra costs for low carbon case studies
Case study | Extra cost per home | % extra uplift on construction cost |
---|---|---|
ML | £5,000 | 2.5% |
EH | £20,000 | 9% |
AH | £36,750 | 18% |
TR | £3,200 | 1.2% |
7.5.2 Energy bills
As noted in Section 6, the main benefit expected from low carbon homes, by both the case study residents’ pre-occupation and the respondents to the wider consumer survey, was lower energy bills. There was a general assumption that such homes would be more energy efficient, leading to lower energy consumption and lower energy bills.
In practice, low carbon did not translate to low energy bills, mainly due to the price of electricity and to performance gap issues. As highlighted in Section 7.1.1, the heat pump systems in the case studies, as designed, were around 3 to 4-and-a-half times more energy efficient in the provision of space heating than a gas boiler (based on the SCoP) although lower in-use efficiencies were inferred using monitoring data. However, the unit cost of electricity was 4 to 5 times higher than gas during the project[footnote 41], and so simply using low carbon heating will not reduce fuel bills. Depending on the in-use performance of a heat pump[footnote 42], it may result in higher energy bills compared with a gas boiler.
Nevertheless, fuel bill savings should be achieved as a result of the addition of other measures: improved building fabric energy efficiency, efficient building services (such as MVHR) and on-site energy generation (such as PV panels), potentially combined with battery storage. Hence it would be expected that the energy bills would be lower in a low carbon home compared to the gas-heated homes that residents may previously have lived in, even if the bills are not as low as might be initially expected.
The SAP predictions of annual space heating and hot water bills for representative units suggest that the performance gap can have a large impact on the energy bills. Developers’ SAP calculations were compared to the research team’s Bf2050-adjusted SAPs including for site observations and fabric testing, in-use performance measurement (for Marmalade Lane flats only) and reported performance issues (for EH only). This is shown in Figure 26. The size of the homes is noted as this varies and impacts on the energy consumption. However, the purpose of the graph is to highlight the difference between the developer-calculated SAP values and those determined by the (Building for 2050) research team, reflecting the impact of the performance gap, rather than the predicted bill in £/m².
Figure 26: Predicted energy costs for space heating and hot water across the case study schemes[footnote 43]
Description of Figure 26:
Bar chart showing the annual predicted energy costs for space heating and hot water across the case study schemes. Details below.
At Active Homes, the specification of electric panel heaters (100% efficiency), coupled with the relatively high unit cost of electricity, resulted in the highest developer SAP predicted bills, despite the smaller floor areas compared to the other case studies. At the other schemes, the higher efficiencies of the heat pumps specified (compared to on-peak electric heating) helps to keep the energy bills lower.
Fabric performance gaps were identified at each of the schemes, which translated into increased predicted energy bills for the Bf2050 adjusted SAP calculations (see also Section 7.2). As highlighted in Table 3 and Table 4, the actual energy consumption was typically greater than the Bf2050 adjusted SAP calculations, which would be expected to further increase energy bills. However, given the relatively small sample size and period of monitoring, more detailed analysis of energy bills from the monitored data is not appropriate.
Many residents did not report that their energy bills were low. While performance issues are further discussed in Section 7.2, some key reasons for this include:
- Energy use was observed for the first heating season. Controls were not optimised as residents learned how to use them and/or found teething issues. For example, with heat pumps, unnecessarily turning up heating (due to cooler radiators), and with complex control systems;
- Performance issues with innovative technologies were picked up in the initial heating season. Some residents were told it would take a year until the systems would be operating efficiently (Active Homes and Etopia Homes). At Etopia Homes, the GSHP system did not work effectively due to on-going issues with the supply of heat from the Earth Energy Bank;
- The energy cost per kWh for electricity being 4 to 5 times higher than the gas equivalent[footnote 44] meaning heat efficiency during colder seasons would need to be 4 to 5 times more efficient to achieve cost parity or for cost savings to be realised. This was particularly an issue at Active Homes where the original design concept of warm air heaters was entirely replaced with electric panel heaters (that is, 100% efficient, so equivalent to a SCoP of 1), although the PV panels with battery storage would have alleviated some of the impact. The PV panels supplied between 6% and 16% of the electricity consumed during the 4-month monitoring period over winter;
- The regular use of on-peak immersion heaters to supply domestic hot water will increase bills. At Active Homes, Etopia Homes and Marmalade Lane, residents could choose to top-up the domestic hot water supply quickly when needed using the back-up on-peak immersion heater (100% efficient), rather than the slower acting but much more efficient heat pump. If residents have sufficient thermal storage, appropriately commissioned systems, and relevant guidance, the use of the immersion heater (and associated costs) can be minimised, while maintaining an adequate domestic hot water supply;
- Renewable energy technology performance typically has high seasonality, and therefore energy bills in low carbon homes will have greater seasonality than bills in standard homes, albeit they may be lower overall. This will be evened out for those making equal monthly payments across the year, but will likely be a challenge for those paying variable monthly bills based on metered use. If residents move in during autumn or winter, their initial bills may be much higher than expected and any monthly payments are likely to be initially determined based on this early use, though they may be revised downwards later;
- The building envelope did not perform as well as designed – even small inefficiencies can lead to a larger performance gap with low carbon heating, as lower flow temperatures lead to increased heating times. While this impact will be lessened through specifying heat pumps rather than direct electric space heating, the key issue is to avoid the performance gap in the first place. This requires detailing to avoid thermal bridging, design co-ordination, better planning for the building services, efficient routing, high standards of workmanship and checking on site, and suitable commissioning. This should be supported by integrated control systems and a comprehensive handover process with on-going support;
- In 3 case studies, there was inadequate stewardship of the systems during commissioning and hand over, resulting in reduced system performance and increased energy bills. At Marmalade Lane residents identified defects with the communal system, and there was a similar situation at Etopia Homes with residents reporting issues;
- There were issues with accessing the intended tariffs at Etopia Homes and Active Homes (time-of-use tariffs were difficult to access, particularly at AH where the dual-phase[footnote 45] supply meant no such tariff was available). For the Marmalade Lane communal system supplying the flats and the ‘Common House’, a higher (commercial) energy tariff was incorrectly applied, and the process of claiming the Renewable Heat Incentive (RHI) was complex, with the application delayed accordingly. Some low carbon home designs (Etopia Homes and Active Homes) fundamentally rely on energy storage and access to time-of-use tariffs to flexibly interact with the wider energy system and keep energy bills low;
- Reducing capital costs through value engineering. This led to the removal of PV from the design in one case (Marmalade Lane) and a change in floor construction at another (Etopia Homes), which adversely affected the performance of the inter-seasonal energy storage.
There was also confusion around the expectations of the size of energy bills and how some of the schemes were marketed. At Active Homes and Etopia Homes, low energy bills were particularly promoted as a key marketing point, but they were not delivered as expected. Some residents also assumed that if there was no gas, and hence no gas bill, their overall energy costs would inherently be lower, without understanding that the heating and hot water loads as well as the cooking would be delivered by electricity, a more expensive fuel.
At Tallack Road, residents had limited awareness of the heating-related component of the service charge, which appears to significantly affect their total heat-related costs. Some felt their energy costs were relatively low without realising that what they paid directly to their supplier was only part of their overall energy costs.
Resident satisfaction was significantly influenced by the expectations set at the decision to rent or purchase stage, and these expectations impacted on trust in the developer when they were not met. Where low energy bills had been used as a key marketing point, the sense of disappointment among residents was heightened. Some case study residents also conducted cost-benefit analysis and decided to pay more for their purchase price/rent to have lower energy bills. Paying more for energy bills than expected had a significant impact on residents’ views towards their homes.
The impact of the performance gap becomes a greater issue for homes with electric heating. Issues with heating system underperformance will have a greater impact on energy bills, given the price of electricity. This was evident for Active Homes and Etopia Homes where the developers were helping to pay residents’ energy bills (in Autumn 2021). In both cases, residents had significant concerns about this arrangement ending with performance issues remaining unresolved. Some Etopia Homes residents also reported being concerned about the impact of technical issues and higher energy bills on their property resale prices.
7.6 Skills, competencies and collaboration
A shortage of knowledge or skills relating to low carbon homes (building fabric detailing and airtightness, technologies, integration, commissioning etc.) presents a challenge to scaling up the delivery of low carbon housing. This includes both a shortage of skilled labour on-site and a lack of capacity in the whole supply chain.
An issue identified in the case studies and wider professional interviews is the greater need for skills and experience of teams involved in the design, as well as the construction, of new low carbon homes. Most stakeholders in the wider interviews believed a shortage of skills presents a challenge to scaling up delivery of low carbon housing. Furthermore, in 3 case studies, residents said developers did not have a good understanding of these homes and systems – a concern when homes were not working as expected. This may lead to negative customer satisfaction and, in the worst case, legal claims regarding underperforming homes.
The case studies demonstrated mixed levels of knowledge and skills from stakeholders, with the level of skill and knowledge directly affecting performance. In general, issues related to M&E design and installation, with less focus on building fabric. That said, a greater focus on avoiding thermal bridging in design and installation (particularly in relation to panelised systems where they abut other non-panelised thermal elements) is needed, as well as on ensuring continuity of the air barrier through service penetrations. Two of the case studies, Active Homes and Marmalade Lane, highlighted a lack of access to sufficiently skilled people in M&E design and installation. In contrast, Tallack Road was an example of having the required M&E design skills (both in-house and through working closely with the manufacturer). In this case, although the system was new to the M&E contractor, the developer provided significant services-focused support.
There was a poor understanding of delivery of the M&E services from design and site teams. For most of the case studies, there was a lack of experience with MVHR systems and with heat pumps. Both are likely to be more prevalent in the future, as low carbon housing designs are adopted. This inexperience resulted in:
- installing MVHR units in cold loft spaces and with lengthy duct runs which reduced the efficiency of the system;
- incorrect commissioning of heat pumps;
- poor commissioning of the MVHR systems;
- issues with location and installation of heat pumps which resulted in noise and vibration, affecting some residents and requiring subsequent relocation.
Furthermore, it was apparent from industry interviews that a lack of continuity of the design team appointments and technical advisors exacerbates this lack of skills, as key knowledge and understanding of the design intent can be lost and design changes can be made with negative consequences.
Gaps identified by the research team included:
- development teams’ lack of holistic understanding of low carbon homes and the potential impacts of decisions;
- not taking advantage of a high-performance fabric to make a saving in the design/sizing of the building services;
- a lack of knowledge and experience on the part of clients, planners and funders, which can negatively affect outcomes at an early stage;
- a need for more knowledge, experience and skills in design, site, and sales teams;
- the mixed availability of skilled labour and training, particularly in relation to MMC and innovative technologies;
- shortages of skilled commissioning engineers, performance evaluators, building control and maintenance staff.
A specific need for additional resources and training for Building Control and Planning Officers was also raised by the wider stakeholder interviews, to help ensure targets are met in practice. In 2 case studies, the local planning authority teams appeared insufficiently experienced to understand and account for the impact on the energy and carbon performance arising from design changes required to address other planning considerations.
A webinar was held in October 2021, with nearly 200 participants, to present the research findings. The research team asked for views using polls, and there were also some open questions. The responses in relation to knowledge, skills, and collaboration are presented below.
Figure 27 shows the types of stakeholders attending. Figure 28, Figure 29 and Figure 30 show feedback on which organisations, in the view of the attendees, require additional knowledge and skills to help deliver or drive demand for low carbon homes at scale. There was a general recognition of the need to increase knowledge and skills across all sectors.
In each case, the bars show the percentage of the total number of respondents selecting each answer.
Figure 27: Organisations represented by attendees at the webinar (response received from 99 of 193 attendees)
Organisation | % |
---|---|
Developer (14 responses) |
14.14% |
Designer / Engineer / Installer (33 responses) |
33.33% |
Government / Local authority (9 responses) |
9.09% |
Energy sector / Technology company / Solutions supplier (37 responses) |
37.37% |
Academic institution (6 responses) |
6.06% |
Figure 28: Whether different parts of the construction sector require additional knowledge and/or skills to deliver low carbon homes at scale. Respondents selected the sectors they thought needed this.
Sector | % |
---|---|
Developers (58 responses) |
95.08% |
Funders of development (43 responses) |
70.49% |
Design teams (57 responses) |
93.44% |
Site teams (55 responses) |
90.16% |
Sales teams (40 responses) |
65.57% |
Figure 29: Whether different organisations require additional knowledge and/or skills to support the delivery of low carbon homes at scale. Respondents selected the bodies they thought needed this.
Description of Figure 29:
Stacked bar chart showing which organisations require additional knowledge and/or skills to support the delivery of low carbon homes at scale. Respondents selected the bodies they thought needed this. There was a general recognition (approx 80%) of the need to increase knowledge and skills across all sectors.
Figure 30: Whether residents, landlords, and lenders require additional knowledge and/or skills to drive the demand for low carbon homes at scale. Respondents selected the groups they thought needed this.
Group | % |
---|---|
Legacy landlords (37 responses) |
68.52% |
Mortgage lenders (41 responses) |
75.93% |
Home owners (43 responses) |
79.63% |
Renters (27 responses) |
50% |
8. Energy System Analysis
The key findings in relation to energy systems:
Low carbon energy sources are being adopted but there are challenges to overcome in terms of optimised operation and associated low energy bills
All the case study schemes had electric heating solutions, with 3 combining this approach with other electric assets such as storage and generation. Managing these assets optimally is important to maintain low energy costs and carbon emissions, but all case studies had operational challenges - as a result of handover processes and the complexity and configuration of controls.
Achieving low carbon, low cost homes will be dependent on their optimum integration with the wider energy system and on efficient end-use operation
It is clear from the case studies that the operational cost and performance outcomes of low carbon developments are not only dependent on the physical design and equipment installed, but also increasingly on how the energy is supplied and how the systems operate. Homes have a role to play in the dynamic nature of the energy system by being smart and flexible and managing and shifting loads.
There is a lack of integration between the construction sector and the energy services and supply sector, and better collaboration is likely to assist the delivery of low carbon homes
The case studies demonstrated a significant shortfall in the housebuilding sector of the emerging offerings from the wider energy sector. With both consumers and behind the meter assets* becoming an important part of energy system optimisation, these 2 sectors can work together to provide an optimal solution. Existing roles and skills do not bridge the gap and a range of new players may be needed to deliver the required services and solutions, and to enable residents to operate low carbon homes effectively.
The electrification of new developments provides a challenge to developers and electricity network companies in understanding the connection requirements. An increased demand for individual properties could require a move away from a single-phase electricity supply, which may have unintended consequences (as at Active Homes**).
*Behind the meter assets are those within the dwelling and so not owned by the utility provider.
**At AHN, the predicted electricity demand was such that the local network operator felt a single-phase electricity supply would be insufficient. Instead, there is a 3-phase supply to each dwelling, with one phase used for the main house, and a second for the kitchen. There is no 3-phase equipment.
The dynamic energy system is not currently reflected in regulatory approaches which means it tends not to be considered by developers and design teams
The current regulatory and compliance structure, in particular modelling of homes using SAP, is not capable of assessing and recognising the operational and dynamic elements of the energy system. This presents a significant barrier to industry best practice, including using designs that recognise and take advantage of the potential benefits of dynamic energy. New solutions to calculating and recognising the dynamic nature of energy supply, and optimised use, are required.
One of the innovative aspects of this project was the inclusion of energy systems experts as part of the research team. They provided wider insights often missed when focusing on the performance of individual dwellings. Furthermore, energy systems and services are a rapidly developing sector due to the drive to meet the UK’s climate change commitments. The learning points below encompass many of the aspects discussed above.
The case studies demonstrate that the operational cost and performance outcomes of low carbon developments are not just dependent on the physical design and the equipment that is installed, but they are increasingly dependent on how the energy is supplied, how the homes operate as part of the broader energy system, and how both will change over time. Two important factors are the varying costs of energy over time (due to fluctuations in the wholesale electricity market) and the varying CO₂ intensity of the electricity grid. The cost and carbon emissions from homes can be reduced if they are designed in a way that can exploit these 2 factors, and therefore support decarbonisation of the broader energy system. This applies while the grid decarbonises, notwithstanding the benefits of delivering energy efficient homes with easy-to-use controls as well[footnote 46]. By including this system-level thinking, 4 key findings were identified which look outside of the traditional development envelope, but which are crucial to understanding how low carbon and low cost homes can be delivered.
Finding 1: Low carbon energy sources are being adopted and low carbon homes will increasingly rely on electricity for their energy needs including heating, and this was the position with all of the case studies
Each of the case study schemes was all-electric and the use of electric heating of various forms is likely to be central for most new low carbon homes. The price differential between electricity and gas means optimisation should be addressed if customers’ bills are to remain manageable, starting from the development planning phase.
This finding needs to be understood in the context of a rapid transition into a smart, flexible, low carbon electricity system. Customers are a dynamic component in the electricity system, which itself can influence the cost and carbon intensity of grid-supplied electricity by shifting and managing loads. An important element of this transition is the emergence of dynamic time-of-use tariffs, and it is likely that such propositions will become more common, if not the norm, in the open market.
Our findings include:
- An electric home is likely to contain a number of components - electricity generation, storage (both thermal[footnote 47] and electricity), and consumption loads - many of which can be operated flexibly to optimise energy usage and aid the balancing of the electricity network. The design of the homes and their services, and the way they are operated, will therefore be integral to the performance of the broader electricity system. All of the case studies used heat pumps (HPs), and solar PV was present at Tallack Road, Active Homes, and Etopia Homes (and on some of the Marmalade Lane homes, they were installed by residents post-completion). Battery storage was used in both Etopia Homes and Active Homes. A successful outcome is dependent on correct optimised operation and all the case study schemes had operational challenges, because of handover processes, the complexity of controls, and/or poor control configuration;
- A technical approach to optimisation was used at Etopia Homes, which should have automated the optimisation with the Smart Home Energy Management (SHEM) systems. However, due to a poorly coordinated design and resulting poor integration between components, the systems did not operate in a coordinated manner, and this was a likely cause of the higher energy loads and costs experienced by residents;
- The schemes with individual HPs (Marmalade Lane and Active Homes) relied on separate controls and occupier operation of the space heating and domestic hot water (DHW) generating systems to operate. This project found that the systems and multiple controls are often too complex for occupiers to be reasonably expected to use optimally, in a coordinated manner. In all the schemes, the systems were much more complex than in a ‘conventional’ new home;
- There is a lack of understanding of electricity distribution network needs. The increased electrification of new developments provides a challenge to developers and electricity network companies in understanding the connection requirements. At Active Homes there were challenges with correctly sizing the network connection and the site-wide distribution, resulting in dual-phase supplies to the homes which may not have been strictly necessary if the diversity had been better understood and managed[footnote 48]. While there is some understanding of low carbon homes within distribution network companies at an innovation level, for example on trial schemes or network innovation projects, most schemes being delivered fall below the ‘innovation radar’ (particularly smaller schemes like Active Homes) and go through the standard connection process. This became evident during an interview with a Western Power Distribution (WPD) innovation team member who was not aware of the Active Homes scheme, but who was involved in a larger housing scheme where alternative approaches to network connections were being trialled;
- None of the schemes were provided with suitable electricity tariff structures or service propositions, negating many of the benefits of electric heating solutions. Enabling residents to switch to a more innovative tariff or service proposition such as a dynamic time-of-use tariff (with an optimised control solution) could help to reduce energy bills, as well as supporting optimisation of local network loads and supply. This can be achieved by householders’ energy consumption at times when prices are low, due to high levels of renewable generation or low demand. This process can potentially be managed by a combination of a home energy management system and integrated/coordinated connected equipment controls if they are installed and calibrated to correctly manage and optimise the different low carbon heating technologies. Clearly, not all domestic energy users will be able to take advantage of time-of-use tariffs.
Finding 2: Achieving low carbon, low cost homes is dependent on their integration with the wider energy system and on efficient end-use operation. This requires consideration of how low carbon homes can have a role in grid balancing, placing the operation and the occupiers at the forefront of the development planning from the outset
All of the case studies included a range of systems and features which should have enabled low carbon and low cost operation, but the outcome was not always successful. The low carbon technologies and controls present in some of the developments could, in theory, enable residents to take advantage of dynamic time-of-use tariffs yielding reduced energy bills, while indirectly also assisting in balancing the grid (albeit at a very small-scale level). Nevertheless, housing developers do not generally consider wider energy systems, mainly due to the lack of awareness of system-wide issues and developments. The Etopia Homes and Active Homes projects had a complex set of systems, but also some of the greatest challenges in trying to be successful. Our findings include:
- The optimal operation from a cost and carbon perspective may need residents to be using a suitable energy service proposition. An emerging solution to enable homes to be operated optimally is the use of innovative energy services (such as engaging a third party). These can help residents better manage low carbon homes, reduce their carbon emissions and running costs, and the third-party can support the wider energy system with grid balancing, as well as allowing customers to benefit from some of the energy system value streams (like income from an ancillary service which can only be accessed through aggregation of loads). Apart from the original plan at Etopia Homes (aggregating and operating the batteries as a virtual power plant), none of the case studies appeared to consider how residents could be supported in accessing these types of services. A simple example is providing access to dynamic time-of-use tariffs with control systems configured to optimise the installed services against the tariff, noting they are offered by suppliers rather than by the Distribution Network Operator (DNO). This could have benefitted all homes with heat pumps and storage, as well as the local electricity network (assuming that local network pricing is somehow reflected in future tariffs), but it was not provided in any of them. While the choice or type of service in a traditional home may have little impact, it could be integral to the performance of a low carbon home and would also offer grid balancing benefits to the local electricity network, but it was not implemented by any of the developers;
- A successful outcome relies on correct operation. This means the design and features of the services need to be correctly specified and residents need to be able to understand how to operate them - like having an overall control scheme that can operate and optimise all the sub-systems. At Marmalade Lane as an example, there were several control systems (MVHR, HP, and 2 heating controllers) for the occupiers, which were challenging to understand individually, and very difficult to optimise in a coordinated manner. Attempts at simplifying this at Etopia Homes with the SHEM were not successful, resulting in an even more challenging and difficult to understand system for occupiers. The development of integrated controls appears to be an opportunity for further innovation;
- Occupiers need to be suitably instructed in the optimal use of the energy systems in their homes. A common finding across all case studies was that residents did not fully understand how to operate their systems following handover. The explanations and training offered were often either too simple or too technical to be of benefit, including the provision of technical manuals (often aimed at installers) rather than home user guides. It was noted that the COVID-19 pandemic impacted on the handover process for 3 of the case studies, but it should not have affected the provision of appropriate guides. This aspect, coming at the end of a project, is often under-resourced. For low carbon homes, bespoke guides and guidance will be needed, with appropriate fees, resources and programme allocated to this, at least for the first few schemes. Follow-up visits may be necessary, beyond the initial handover, and advice should be provided when seasons change (for example, how to minimise overheating risks).
Finding 3: There is a lack of integration between the construction sector and the energy services and supply sector, with this large gap hindering the delivery of low carbon, low cost homes
While developers are being charged with delivering low carbon, low cost housing, many of the potential solutions and innovations arise from the energy sector – utilities, appliance and control manufacturer companies, and innovative business model/service providers.
The culture and skills in these 2 sectors are different and it is important that they can better align and work together, understanding the solutions that each can provide. Our findings include:
- A good understanding of the energy system and ongoing changes is outside of the expertise of housing developers who are primarily focused on the physical build process. This could be partly addressed through the inclusion of an energy expert within the design team, and early engagement with energy services providers (as distinct from utility providers);
- None of the case studies demonstrated a robust understanding or integration of energy system needs into the design and implementation of the scheme. Perhaps the most advanced scheme was Etopia Homes with the initial inclusion of a community energy microgrid scheme with smart operation, developed by a specialist consultant who was part of the design team. The early concepts appeared to be consistent with innovation in the energy sector, but the reduced role - and then exit - of the specialist consultant, resulted in a more conventional approach that did not align with the original design. There could be a role for organisations including suitably skilled local authorities and/or commercial service providers to create and manage community energy schemes;
- Active Homes was initially intending to have a communal battery, but this was changed to individual batteries by the housing association. The primary reason for this change was that to operate a communal battery, the housing association would have needed to become an energy provider, with associated practical and regulatory implications[footnote 49]. The other reason cited was that there could be issues with social cohesion at the new development, due to the risk of a perception that some residents benefitted from the battery storage system more than others. Because the scheme was approached from a technological perspective, there were no identifiable solutions to overcome this, although it is an issue common to lots of local energy systems. A number of innovators are exploring business models and customer propositions to attempt to address the equality issue;
- There are a range of specialists focussing on providing low carbon service propositions for new homes at an innovation or ‘early market’ stage. However, they fall outside of the developers’ usual knowledge area and supply chain. Industry needs to open up to consider and adopt a much broader set of solutions and offerings. This is likely to challenge existing procurement and development process models and will require a change to the make-up of design teams and advisors, as noted above;
- The lack of understanding and experience about energy technologies and service offerings means that developers may, with all good intentions, adopt inappropriate or untried solutions developed by organisations who do not have a suitable understanding of delivering homes. In Active Homes, this was evident with the early ‘homes as power stations’ concept and academic-led design approach.
The government’s announcement that sales of new petrol and diesel cars and vans must end in the UK by 2030[footnote 50] was followed by the addition of Part S to the Building Regulations, requiring that from 2022, all new homes with parking spaces need to have electric vehicle (EV) charging points. The wide-scale uptake of EVs provides a potential additional demand for electricity within homes (most likely in the evenings and overnight). This begs wider consideration of time-of-use tariffs. It could add to the electricity load of a property, challenging the limited capacity within existing sub-stations and driving the need for flexibility solutions, which can help manage and shift loads to mitigate peaks on the network.
Finding 4: The dynamic energy system is not currently reflected in regulatory approaches and the existing methods for designing and accounting for cost and carbon are not appropriate for low carbon homes
The traditional development model detaches design and build costs from operational costs, but the findings from the case studies demonstrate the need to consider the design and build and operational stages coherently. In addition, the existing approaches to calculating carbon emissions and operational costs, which treat the home with a simple boundary, are no longer appropriate and are unable to account for the variability and flexibility in energy supply and demand. Our findings include:
- The regulatory frameworks, in particular the Building Regulations and SAP, are buildings-focused and take a very direct and boundary-focused view of the building and its operation. They do not consider externalities such as the dynamic nature of the energy system, in particular electricity supply. Given the significant variation in cost and carbon emissions of electricity generation across time, it is impossible for regulatory frameworks to represent the actual cost and carbon emissions from homes under the current structure. The Building Regulations need to evolve to include the dynamic nature of electricity supply, and therefore the significant variation in performance which may be achieved using different control strategies, optimisation methods, energy service business models, and time-of-use tariffs. Regulations form a central part of the design process, and if these elements are not included (drawing on associated standards such as PAS 1878[footnote 51]), then they are effectively still ‘off-radar’ for most developers with no value placed on including them;
- Another important finding from the research (and linked to the dynamic nature of the energy system) is that energy kWh, CO₂, and cost are no longer proportional. In the existing Building Regulations relating to energy use and conservation, the focus is on reducing energy and CO₂ (they are assumed to be proportional in SAP), with a corresponding reduction in cost. However, the dynamic CO₂ intensity and cost of energy means that lower carbon and lower cost outcomes (such as heating) may be achieved with higher levels of energy consumption, such as through more continuous heat pump operation with off-peak thermal store charging. This means that an assessment approach is needed that does not assume energy consumption, carbon emissions, and cost, are all proportional. While not directly observable in the case studies, this is a critically important point in understanding many operational and optimisation findings from them. It is inherent in the energy transition and forms a foundation for many of the report’s findings;
- The case studies identified that new forms of infrastructure may also be required, such as communal heating systems (Tallack Road), ground loops for heat pumps, (at Etopia Homes) or microgrids (considered early on at Etopia Homes but not implemented). All of these can result in a high upfront development cost. However, the project research raised the potential unfairness of the funding model when these innovative infrastructure solutions (which may result in a lower overall energy system cost) are compared with the traditional regulated electricity and gas network infrastructure, which is funded through ongoing network charges on energy bills. This means that developers (and house buyers) are paying an up-front premium for low carbon infrastructure, but not for (potentially higher carbon) traditional infrastructure approaches. There may be a need to provide new regulatory structures to fund low carbon infrastructure, promoting low carbon solution business models.
9. Summary of key findings
No major housebuilder was delivering low carbon homes at scale where a planning policy driver is not in place (Autumn 2021[footnote 52]). This was demonstrated through the selected case study schemes. The initial intention was to review 3 or 4 low carbon developments being delivered by major housebuilders to determine what was driving them, what they were delivering, and how the homes were performing, but no such examples were identified or put forward. Instead, the schemes that were selected in 2017 and 2018 were undertaken by SME developers (Marmalade Lane and Etopia Homes); in response to a planning driver and desire to test innovation (Tallack Road); or because of a desire to address fuel poverty and climate change, embrace innovation and support local employment (social landlord, Active Homes).
As regulatory drivers, Building Regulations Part L 2021, which came into force in England on 15 June 2022, and the forthcoming Future Homes Standard due in 2025, will drive both higher energy efficiency standards and consideration of low carbon forms of heating which reflect the updated carbon factors.
However, to ensure the government will meet its target for the UK to be net zero by 2050, it is essential that the new homes planned and delivered now, and in the future, will not need a significant upgrade by 2050. This requires many more low carbon homes to be built and they must perform as expected; a sharp focus on low cost, optimised designs and delivery is needed.
The key findings that emerge from looking across the data sources and analysis reported in Sections 5 to 8 are set out in Table 7. For ease of presentation, the findings are divided into two: (i) those relating to supply and demand, and (ii) those relating to low energy and low carbon performance. The key learning points arising from these findings are discussed in Section 10 and are presented in the same 2 categories.
Table 7: Key findings
Supply and Demand
1. The main barriers for developers and housebuilders expressed through the stakeholder interviews (see Section 5.4) are perceived additional costs, a reluctance to change existing delivery models, and a lack of clarity over the long-term changes required to deliver net zero carbon homes. There are other barriers around insufficient energy infrastructure, the use of innovative approaches, and a perception that customers will have a negative attitude to innovative technologies and homes.
2. The drivers for low carbon homes have been identified – for developers and for residents (see Section 5.2 and Section 6.2 respectively).
All industry stakeholders said that to deliver low carbon homes at scale, national regulation is necessary to set a higher minimum performance standard.
In advance of this, and potentially at a local or organisational level, other drivers apply:
- A local vision for low carbon homes and an increased awareness of climate emergency;
- Local planning policy;
- A desire to deliver lower running costs and improved comfort. This was stated as a benefit of low carbon homes by all supply-side case study stakeholders;
- A desire to support brand and product development to address low or zero carbon targets.
There is now a much greater awareness of climate change and the need to respond amongst both professionals and the public.
The interest from the public relates to being attracted to low carbon homes, making use of renewable energy, and reducing their environmental impacts. They also have the expectation of lower running costs. Notably, 11% of respondents from the wider survey said they would be prepared to pay more for a low carbon home.
However, as climate change concerns are not the primary driver when selecting a home, on its own this interest will not be sufficient to drive a significant increase in the supply. Other actions will be needed to drive the market.
This could include using both local authority influences and public sector finance to drive the market in advance of higher regulatory standards.
The lack of availability of low carbon homes in areas where people wish to live, and the lack of information regarding low carbon homes, were cited as barriers by consumers.
3. For developers, the additional capital cost required to design and construct low carbon homes need not be a significant barrier to delivery, as demonstrated by the reported additional capital cost for 2 schemes of only 1% to 2.5% of the construction cost (see Section 7.5.1).
Two schemes had much higher additional costs, at 18% (Active Homes) and 9% (Etopia Homes). These schemes received grant funding and were intentionally more innovative. In practice, these complex designs were challenging to deliver and the performance fell short of expectations. Both developers have since adopted simpler, lower-cost solutions.
Interviews with the housing industry confirmed that a small increase in construction costs would not prevent homes being built, as the land and development market would adjust through changing sales values, land value and improved supply chains (see Section 5.4).
4. Developers and wider industry stakeholders noted that insufficient supporting energy infrastructure can be a barrier (see Section 5.4). The issues raised included the lack of capacity in the local electricity grids for fully electric homes and upgrade costs being borne by developers, despite them not owning or having guaranteed access to the upgraded infrastructure.
Compared with the traditional regulated electricity and gas network infrastructure, which is funded through ongoing network charges on energy bills, innovative infrastructure solutions (which may result in a lower overall energy system cost) are funded through developers, and house buyers, paying for them up-front. This can be addressed through working closely with energy service providers, or at a national level through new business models and new regulatory structures to fund low carbon infrastructure, or both (see Section 8).
5. Lack of access to private finance for early adopters may not be a significant barrier as real estate investors develop and implement their ESG strategies.
The increased availability of funding was cited by stakeholders as a more recent (2021 onwards) enabler for low carbon housing and is supported by changes to the Sustainable Finance Disclosure Regulations.
All wider stakeholders working in real estate investment and development stated that there was now (in 2021) much more finance available to develop low carbon housing compared to a few years ago (see Section 5.3).
6. Lower running costs were cited as a key driver for residents and reducing fuel poverty is a key driver for some developers, especially social landlords (see Sections 6.3 and 6.6 – residents and Section 5.2 – landlords).
Furthermore, in the wider survey (forced choice experiment), 44% stated they would be prepared to pay more for a low carbon home on the expectation of lower running costs, further reducing the barrier of additional capital costs (Section 6.3).
When asked about benefits and challenges of sustainable homes, 58% expected lower energy bills to be a benefit, and 13% expected higher energy bills to be a challenge (wider survey).
7. A lower environmental impact from their home and/or using a renewable source of energy is a principal driver for residents/potential residents (especially some demographic groups) as it supports their personal values, but most said that their interest was mainly financially driven in terms of having lower energy bills (see Section 6.3).
As noted above, in the wider survey, forced choice responses showed 44% always chose the sustainable home regardless of cost (it should be noted that this was always accompanied by at least 50% saving in energy bills), 14% always chose the traditional home, and 42% sometimes selected sustainable homes and other times selected traditional depending on cost and other attributes, demonstrating support for sustainable homes.
8. The biggest barrier perceived by potential residents (not the case study residents) is that low carbon homes will cost more. However, the dwellings in the case study schemes were sold at market rate and not at a premium cost, noting some developers took a reduction in profit or paid slightly less for the land (see Section 6.4).
The case studies demonstrate the potential for only a minor construction cost increase for low carbon homes at scale and observed sales values that were not increased above those for standard, non-low carbon homes (see Section 7.5.1).
9. A lack of availability of low carbon homes, especially in the areas residents wish to live, is a key barrier that was reported in the wider consumer survey (see Section 6.4).
Many of the case study residents felt there is a growing demand for sustainable homes, but very few were aware of other developments with sustainable homes and felt that such homes were largely confined to those who choose to build their own properties. This could be an issue of awareness, availability, or both (see Section 6.4).
10. For those with fewer constraints on location, or more driven by sustainability concerns, there is no easy way to identify where low carbon homes (rather than standard new homes) are being built (see Section 6.4).
At least one case study resident suggested there should be more information online, via property search websites, about the low carbon/environmental performance of a home, and a number of aspects that could be filtered within searches (such as solar panels).
11. When the research team created a Bf2050-adjusted SAP (based on observations and testing) and compared the resulting EPC with that generated from the developer-calculated SAP, the EPC for the Bf2050-selected sample dwelling remained the same for 3 out of 4 case studies. This demonstrates the lack of granularity in the EPC rating (see Section 7.3 and Table 5).
This lack of granularity means that those developers targeting exceptional carbon performance cannot differentiate themselves from others who are targeting an EPC ‘A’ rating via a lower specification.
This is also a barrier for those looking to buy homes with exceptional low carbon performance, as these cannot be easily identified. The exception is Passivhaus certification, which is a recognised low carbon performance standard that also takes overheating risk into account.
12. The public sector can play a significant role in promoting more low carbon homes through setting performance requirements.
This was noted as a driver (see Section 5.2), typically led by the local authority, affordable housing provider, or local community group. The low carbon performance targets were influenced by the local authority in all of the case studies. In 3 cases, the local authority was the original landowner, setting the brief for low carbon homes on these sites, and at the fourth, the influence was via planning policy.
Further examples were given in stakeholder interviews who highlighted local authorities such as Cambridge, Oxfordshire, Central Lincolnshire, Cornwall Council, Exeter, Norwich, and some London boroughs who are developing and funding homes that meet improved low carbon standards such as Passivhaus or Net Zero Carbon. These local authorities have successfully driven the market in advance of Building Regulations, and most stakeholders noted that this should be scaled up across the public sector, with a consistent approach to affordable housing funding requirements.
Local authorities are responding to the climate change concerns of councillors and voters, and some also recognise the opportunity for local green employment and investment associated with low carbon homes. However, national housebuilders who wish to be able to deliver the same designs across all of their developments are not in favour of localised requirements.
13. The research activities identify an increased high-level awareness of climate change, when compared to the start of the project and literature review of 2019. This increased awareness was witnessed across both the case studies and the wider technical interviews, with an associated drive for an improved carbon standard for housing (see Sections 5.2 and 6.3).
The increased awareness of the need to act was witnessed both at an organisational and personal level across funders, clients, design teams and on site. It is not confined to local authorities: leading developers and some SMEs have made their own commitments in relation to climate change and becoming net zero carbon and are acting accordingly. Some investment funds are also recognising the climate change imperative and are looking to target their investments accordingly.
14. This research has focused on low carbon homes, not zero carbon homes. Any additional upfront cost to deliver low carbon homes will need to be considered in the context of the cost of future retrofit if a net zero home is not delivered, particularly in relation to building fabric upgrades. These future upgrade costs will be borne by the landlord or homeowner, and not by the developer.
Energy and Carbon Performance
15. The updated Part L of the Building Regulations in both England and Wales will drive the requirement for lower carbon homes. However, based on the performance monitoring in Section 7.2, without additional focus, there is a risk that homes built to these updated regulations will not perform in use as developers and designers intend, or as residents hope for.
The performance of low carbon homes that are delivered needs to be improved, which will assist in reducing energy bills. Residents also need greater consideration – carbon emissions are not the only issue that matters.
16. As seen in Section 7.2 that when low carbon homes are constructed, there can be a significant performance gap between design and performance. This performance gap could be mitigated with better upfront design and stronger integration across disciplines from the start. Initially, at least, a low carbon advisor may need to be added to the team and retained through to practical completion.
The performance gap for a heat pump system is relatively more significant in terms of increased energy demand and the impact on electricity use and running costs, compared to traditional new build homes using gas for heating and hot water. This was apparent through comparisons between the developers’ as-built SAP calculations and those produced by the research team, taking the measured values, site testing, and site observations into account.
Site teams do not currently understand the importance of close attention to detail with the change to constructing low carbon homes. Examples of the checks required include ensuring there is no missing insulation, addressing thermal bridging risks, ensuring airtightness standards are delivered, and the correct commissioning of equipment. Handover advice is also a key part of closing the performance gap, in relation to occupant understanding and behaviour.
Developers, design teams, and site-based teams need to understand why and how to ensure as-designed performance is delivered.
17. The UK’s Net Zero Carbon commitment by 2050 (and interim target by 2035) will require additional considerations, beyond carbon emissions from regulated operational energy at the design and as-built stages. Case study analysis (Section 7) and stakeholder interviews (Section 5) suggested that additional considerations should include embodied carbon, the assessment of performance in-use, recognition of the dynamic carbon intensity of grid-supplied electricity[footnote 53], and wider measures for energy infrastructure upgrades to address the demands of new all-electric homes.
18. As shown in Section 7.6, the case studies and the wider professional interviews identified a greater need for skills and experience by the teams involved in the design of new low carbon homes, as well as those constructing them. In general, the issues related to M&E design and installation, particularly around MVHR systems and heat pumps, technologies which are likely to become more prevalent. In terms of the building fabric, a greater focus is also needed on avoiding thermal bridging, and on avoiding disrupting the continuity of the air barrier through service penetrations.
A specific need for additional resources and training for Building Control and Planning Officers was also raised by the wider technical stakeholder interviews, to help ensure targets are met in practice.
19. Developers and design teams need to think more broadly than just delivering low carbon homes, otherwise there is a risk of unintended consequences. Other key considerations include buildability, compatibility of components, lower running costs, usability, plant locations, controls integration, and comfort in all seasons.
The unintended consequences can be higher energy bills, higher embodied carbon, challenges with maintenance, over or under ventilation, and overheating. As an example from Section 7, at some of the case study schemes, the focus on low carbon resulted in more complex solutions which over-rode considerations of ease of operation.
20. Residents reported they had insufficient hot water at 3 of the 4 case study sites (see Section 6.5). This is likely to reflect comparison with instantaneous hot water supplies in previous homes (from gas combination boilers) in some cases. Whether a consequence of availability issues or not, much lower than predicted hot water consumption was observed.[footnote 54]
The poorer availability was likely to be due to a combination of factors – the lower temperature delivered by heat pumps, the DHW cylinder size, slower recharge rates than for higher temperature hot water generating systems, and whether an immersion heater boost was available.
Designers need to ensure low carbon homes are not associated with insufficient hot water.
21. Warmth, comfort, and good indoor air quality were expected benefits for residents and were delivered (see Section 6).
Improved commissioning should address the ‘dry air’ reported by some, and improved system design would overcome the cold down draughts experienced at another case study.
The warm overnight temperatures in bedrooms in winter were reported as a hard to get used to by a few residents (those used to sleeping at lower temperatures).
22. Some residents were unhappy with the constraints on their behaviour that living in their low carbon home imposed (see Section 6) and felt they should have been warned about them, prior to moving in. As examples, in one case study the heating switched off if the doors or windows were left open, and at another case study, the heating was limited to a maximum of 22oC. Furthermore, some residents felt they had to adjust their behaviour to match the lower DHW availability.
Some residents had difficulty understanding the benefits from a change in behaviour. Some struggled to accept there was no need to open windows for fresh air since they had an MVHR unit, and instead maintained their previous window opening habits.
23. As shown in Section 6, 3 of the case studies reported overheating during the summer. This improved over the course of occupancy as residents got used to using their systems and implemented solar shading solutions or window-opening routines.
It is noted that the introduction of Part O of the Building Regulations should better ensure that building designs minimise the risk of overheating.
Developers need to be clear in their handovers and written advice around window opening behaviour. Some residents thought the advice to avoid opening windows because their dwellings had MVHR units applied all year-round.
Two out of 4 developers are considering providing cooling using ASHPs at future schemes. As highlighted in the guidance accompanying Part O, passive design approaches, rather than active approaches, are preferable in terms of lower carbon emissions and running costs. It also seems counterintuitive that low carbon homes need to have active cooling.
24. As shown in Section 7 and Section 8, a move to low carbon heating means that energy consumption, CO₂, and cost are no longer directly proportional. A dwelling can be lower energy and lower carbon due to the use of a heat pump but can have higher energy costs than a gas heating system due to the higher unit cost of electricity compared to gas.
Marketing homes as having low bills is likely to attract future residents, as it was demonstrated as one of the main perceived benefits that such homes will deliver in Section 6. However, the energy bills across the case study schemes were not as low as expected. Some residents experienced bills that were significantly higher than they expected and were concerned about their ability to pay them.
Section 8 shows that low carbon homes are likely to be heavily electrified. Achieving low costs and low carbon emissions will require optimised control, combined with suitable tariffs and service propositions. In practice, the increased complexity means that a degree of automation will be necessary to enable consumers to manage the systems, or the operation needs to be outsourced to a service company.
25. At each case study, there was a significant performance gap (building fabric, services, and commissioning) and/or overly complex building services designs that led to increased running costs (see Section 7). It is important that residents have confidence in their ability to operate their systems to meet their needs for affordable energy bills.
Another key contributor to these higher running costs was the significant difference in unit costs of gas vs electricity, which was not negated by the improved efficiency of space heating and hot water generation. The planned access to lower cost electricity tariffs at some case studies was also not achieved in practice.
The higher cost for a unit of grid-based electricity compared to a unit of gas (4 to 5 times higher when the research project was concluding[footnote 55]) is hard to overcome through increased plant efficiencies and renewable energy generation. This is an important learning point in relation to the retrofit of low carbon heating systems as well.
In addition to reducing running costs through fabric measures, on-site generation, battery or inter-seasonal storage, and lower cost tariffs can all play a role. There were some performance issues relating to this in the case studies, which should be avoidable at future developments.
Delivering lower energy costs needs to be a greater focus for developers, designers, and site teams during design and construction to ensure the performance gap is closed. This should be continued during the handover process to ensure good occupant understanding of systems and controls, and appropriate behaviour. This is because low energy bills are the greatest driver for residents, and concerns around the complexity and performance of, and need for interaction with, low carbon technologies are one of the greatest barriers (See Section 6).
There is a role for regulators to ensure low carbon homes will deliver low energy costs too, and that the low carbon assets such as heat pumps are operated in an optimal manner with suitable tariffs and/or service models in place (see Section 8). This may require an operational assessment of performance.
26. The case studies demonstrate that the operational cost and performance outcomes of low carbon developments are not solely dependent on the physical design and the equipment installed. Increasingly they also depend on how the energy is supplied and how the homes operate as part of the broader energy system (see Section 8).
Two important factors are the varying costs of energy over a 24-hour period and the varying CO₂ intensity of the electricity grid (daily and seasonally). The cost and carbon emissions from homes can be reduced if they are designed in a way that can exploit these 2 factors, and they therefore support decarbonisation of the broader energy system.
27. There is a significant shortfall in knowledge amongst the housing sector about developments within, and offerings from, the energy sector (see Section 8). This needs to be addressed to ensure the best outcomes for residents of low carbon homes.
While developers are being charged with delivering low carbon low cost housing, many of the potential solutions and innovations are arising from the energy sector: utilities, appliance and controls manufacturers, and innovative business model/service providers. The culture and skills in these 2 sectors are different and it is important that they can better align and work together, understanding the solutions that each can provide.
28. Some innovative features from the case studies could not be applied in the SAP models, either as standalone technologies or in the combination in which they were used. As such they did not receive the likely full carbon benefit – noting that this was SAP 2012 (see Section 7.1).
While SAP is a compliance tool, not a design tool, designs often focus on what is more cost-effective to achieve compliance with SAP. Not being able to easily select a technology was reported by some as a barrier to innovation. This is being considered for SAP 11. However, it is important to ensure that the SAP methodology is robust, which can impact on how more innovative technologies are represented, in terms of assumptions and the process of applying for inclusion in SAP.
29. Energy storage has the potential to allow residents to maximise the use of energy generated on-site across a development or at an individual dwelling level (see Section 8). This can be achieved through individual dwelling-level batteries, or a communal system (as originally proposed at Active Homes).
Storage requires optimised operation through a combination of (or use of all) automated controls, home energy management systems, and suitable tariff provisions, to allow exploitation of the on-site balancing and imported electricity costs. This was not achieved on any of the sites due to insufficient consideration around the operational phases.
Communal storage systems also require some form of microgrid. The original proposal for a communal battery at Active Homes was partially changed to individual dwelling systems due to lack of a communal control strategy and the challenges in sharing the value from the battery amongst residents.
Storage can also present a challenge for the DNO connection and can (depending on how they are connected at a dwelling level) present an additional load, which could incur a larger and more expensive network connection. This is due to a lack of understanding or established practice on how to consider aggregated loads across electric assets at new developments. This was another reason a communal battery at Active Homes (seen by the DNO as a separate load) was changed to individual dwelling batteries (which are considered part of the dwelling load).
As highlighted throughout this report, there are also challenges in relation to storage in the regulatory framework, and SAP is currently not able to adequately assess or recognise the dynamic benefits of storage.
10. Next steps
10.1 Introduction
Building for 2050 was commissioned to understand the drivers and barriers to the large-scale construction and take-up of low cost, low carbon housing. The research team was tasked with identifying approaches to minimise cost premiums, improve energy efficiency, reduce carbon emissions, increase consumer demand, and accelerate industry delivery. Like the main findings set out in Section 9, this section, which highlights key learning points, is arranged into 2 distinct groups:
- Learning points that address supply and demand (Section 10.2.1)
- Learning points that address energy and carbon performance (Section 10.2.2)
In essence, these are the next steps the UK construction industry should take in its bid to deliver – and see the take up – of low carbon homes at scale. The new homes currently being designed and delivered are not in line with the UK government’s Net Zero Carbon commitment.
The COVID-19 pandemic was evident during the research project, and the construction industry also needed to adjust to the impacts of Brexit. Nevertheless, these learning points are considered appropriate and relevant in the context of the need to accelerate delivery of low carbon homes in general.
10.2 The key learning points in detail
10.2.1 A discussion on increasing supply and demand
Actions are needed to increase the supply of low carbon homes, while at the same time addressing the capital cost uplift, on-site delivery, and performance in use.
The demand for low carbon homes has been demonstrated by case study residents and a consumer survey, which noted:
- there is a presumption that low carbon homes will deliver low energy bills;
- addressing environmental concerns is another driver, including the use of renewable energy technologies.
There are also a number of barriers to purchasing or renting low carbon homes, primarily:
- a perception that such homes will cost more;
- insufficient knowledge about low carbon homes – their location and intended performance;
- concerns about the performance and reliability of homes and technology;
- the challenge of learning about new technology and how to use the systems;
- concerns about overheating.
Overcoming these barriers will take a multifaceted approach and includes: having a roadmap, clarity regarding the future scope of regulations, adopting a new approach to design, gaining a better understanding of new products and offerings from the energy services sector, and sharing of experience as part of a wider upskilling programme.
Taking innovative funding approaches and simplifying design and optimisation should drive greater uptake of low carbon homes by reducing the capital cost barrier - but investment will be needed. This should be targeted at low carbon design advice, design integration, upskilling, quality assurance of site practices, and reducing snagging issues, and there will be learning through experience (across the supply chain). Furthermore, as low carbon products and specialist contractors become integrated within supply chains, cost advantages should start to be realised.
It is essential that low carbon homes perform well and deliver low energy bills, boost consumer confidence, and that future residents’ concerns are allayed. If the primary driver for residents of low (not just lower) energy bills is not delivered in practice, this will send negative signals to the market – both those seeking to deliver low carbon homes and those planning to live in them.
Seven key learning points have been identified relating to increasing the supply of and demand for low carbon homes:
-
Industry groups are requesting certainty on future requirements for low carbon homes - the scope and timing of regulatory requirements and clarity on the role of planning policy.
-
Local planning policy is a key driver for lower carbon homes.
-
The public sector can play a significant role in promoting more low carbon homes by setting performance requirements.
-
In well-planned, well-constructed low carbon developments, the capital cost uplift is minimal and not a deterrent to delivery. Wider uptake would be likely to further reduce this uplift. Highly complex designs aiming to deliver zero carbon or energy positive homes are likely to have on-costs considerably more than the market will pay for.
-
Counter to consumer perceptions, case study homes were no more costly to buy than standard homes (based on the low carbon features), but the broader issue of insufficient low carbon homes and easy-to-access information about them remains problematic.
-
There is likely to be an increased demand for low carbon homes if developers market them with details of features and running costs and provide post-occupancy support to ensure they perform as promised.
-
The construction sector and the energy services sector need to better engage. This will support delivery of low carbon homes, while avoiding significant energy infrastructure costs.
These points are further discussed below.
1. Industry groups are requesting certainty on future requirements for low carbon homes - the scope and timing of regulatory requirements and clarity on the role of planning policy.
In case study and wider industry stakeholder interviews there was a common call for a clear, detailed, government-backed roadmap outlining future requirements in relation to low carbon homes. Any roadmap will need to set out the scope and timing of new requirements and identify the role of planning policy at local level to set more ambitious standards.
Most feedback was received in advance of, or during, the government’s consultation on Part L 2021. This will have been somewhat addressed by the consultation response, which provided a good steer on what the overall performance standard (notional building) will be for the Future Homes Standard in 2025.
There are key elements of the Future Homes Standard currently in development that could impact on industry preparations. The consultation proposes the extension of performance testing to include more than airtightness testing (which the research team agrees with) but does not yet provide details on what this testing regime would comprise. Developers will need time to assess how their buildings perform against any new test methods, which in turn could impact on how schemes and the individual dwellings are designed and delivered, the technologies used, and the training provided.
Stakeholders also requested a need for longer-term certainty, meaning changes in the wake of the Future Homes Standard. One example of this is any future requirement around whole life carbon assessment, as the current focus in the Building Regulations is regulated operational carbon emissions only. Again, housebuilder responses to this requirement will impact on design, building material and technology selection, delivery, and training.
Such longer-term requirements are particularly relevant for:
- those undertaking masterplanning projects, which comprise large schemes that will be built out over many years;
- those investing in upgrades to energy infrastructure to allow longer-term planning;
- those investing in new products and approaches to deliver low carbon homes, including the means of determining performance prior to handover;
- longer-term planning for the upskilling/reskilling of those in the construction industry, across the whole spectrum of services.
2. Local planning policy is a key driver for lower carbon homes
Local authorities have a significant role to play; directly as landowners setting a low carbon development brief, and indirectly as a planning authority setting requirements in policy. In 3 out of 4 case studies, a local planning policy with higher standards than the Building Regulations was driving the development.
Beyond the case studies, wider industry stakeholders noted examples of local authorities who set planning policies higher than regulatory standards. Some of these stakeholders noted that local planning authorities (LPAs) may not have the resources to develop and apply such policies, meaning this driver cannot be acted upon. Adopting such a policy requires suitably skilled and fully resourced development planning teams to set the policy, informed planning officers to determine applications[footnote 56], and sufficient staffing in building control bodies to ensure compliance[footnote 57]. Wider industry stakeholders agreed that further training and resources are required to ensure planners are sufficiently skilled and understand that significant design changes may reduce the overall performance (energy, carbon, running costs, comfort) or increase capital costs, and who take this in to account in their decision-making.
In addition to setting carbon and renewable energy targets to achieve at the design stage, LPAs may also set planning conditions regarding the calculation of embodied carbon, as-built performance, and post-occupancy evaluation (POE). One stakeholder suggested Milton Keynes Council as an example of an LPA setting a POE requirement. Another stakeholder recommended that the new British Standard on Building Performance Evaluation (BS 40101[footnote 58]) could be required by local authorities, and this could help drive greater performance and learning across the sector.
3. The public sector can play a significant role in promoting more low carbon homes by setting performance requirements
All the case studies responded to a local vision, with the public sector landowner requiring homes to meet low carbon standards in advance of updated Building Regulations coming into force. This was a core driver behind all the case studies, either direct involvement as landowner and funder at Neath and Cambridge, or indirectly through the local plan in Corby and London. The literature review and industry stakeholder interviews highlighted that this driver is not commonly present in all public sector land being developed for housing. There is the clear potential for the whole public sector to promote, invest and deliver more low carbon homes.
4. In well-planned, well-constructed low carbon developments, the capital cost uplift is minimal and not a deterrent to delivery. Wider uptake would be likely to further reduce this uplift. Highly complex designs aiming to deliver zero carbon or energy positive homes are likely to have on-costs considerably more than the market will pay for; grant funding was received by the 2 schemes with these more ambitious aims
For developers, the additional capital cost required to design and construct low carbon homes[footnote 59] need not be a significant barrier to delivery. Furthermore, any increased sales price should be set against upgrade costs landlords or homeowners will be required to make to meet the UK government’s 2050 net zero target if their homes fall short of compliance.
Case study and wider technical stakeholder interviews noted a cost premium associated with the inclusion of low carbon measures. The cost data for the case studies shows this holds true in practice. However, it is not as significant as these wider stakeholders reported, and it did not
impact the sales price to residents. Rather, the increase in construction cost has reduced the amount of profit each developer has made in 2 cases (those without grant funding – Marmalade Lane and Tallack Road). So, while there is a perception of increased cost of these homes, the reality is that this did not impact sales prices in these case studies.
For the 2 case studies without an external source of funding, the additional capital cost was only 1% to 2.5% of the construction cost and this did not impact on sales prices. For some of the case studies, developing their brand and offer was a strong driver.
Each of the case study schemes used some new products, or at least new to the UK market. All of them are applying a simpler, lower-cost solution in future schemes. Furthermore, the case study teams expect further product development to better align with market needs. Some of the off-site manufacturers intended to offer a greater size range of offsite manufactured panelised solutions and offer the off-site construction of other building fabric elements.
The research team recommends that focus is required to minimise additional capital through optimising the site layout and form factor, design optimisation (the fabric and services in combination) and innovative procurement approaches. Further cost reductions are likely to be realised as savings are achieved by an expanded supply chain and greater familiarisation from experienced development teams. Profit levels should then return.
Furthermore, the research team considers that while further grant funding might be appropriate, it needs to ensure early schemes are not effectively ‘pilots’ resulting in techno-heavy designs and potentially a sub-standard experience for residents. Such funding is better targeted at expertise to support a project across all stages of construction, through to the handover (rather than meeting the costs of kit). Without a continuity of advice, there is a risk that changes are made which compromise performance due to a lack of understanding of the design intent.
Capital cost increases can be mitigated in several ways:
- For early/pilot schemes, an innovative procurement approach, including partnering with suppliers, can reduce capital costs incurred during this learning phase. As an example, a joint venture was formed between TOWN and Trivselhus, at Marmalade Lane. A Trivselhus timber panel product was nominated for use by the contractor to showcase it to the UK market;
- Adopting passive design principles at the outset, so that rooms and roofs benefit from winter-time solar gains (with planners’ support), such as the sawtooth roof designs at Marmalade Lane to facilitate solar PV panels. Active Homes had to change some dwellings away from the optimum passive solar orientation, reducing performance and increasing capital costs to increase the extent of the TSC area;
- Focusing on performance optimisation, rather than an aspirational target requiring innovative technologies. Pobl and Etopia have simplified their designs at later schemes, adopting the technologies that were most cost-effective in earlier schemes to deliver carbon savings;
- Adopting a fabric first approach to reduce the space heating demand and ensuring the building services are correctly sized, not over-specified or overly complex. The low carbon heating system is likely to have a greater capital cost than a gas boiler, so reducing the demand reduces the plant size and additional costs;
- The heating system should be correctly sized, planning for diversity of loads etc. In comparison with gas boilers, it is more expensive to oversize low carbon heating systems. This can also deliver benefits in terms of reduced sub-station costs (lower additional costs) and avoid the need for dual-phase electricity supplies. The role of battery or thermal storage needs to be carefully considered in reducing running costs;
- Investing in early-stage low carbon expert advice to ensure the design is optimised to both minimise cost and deliver low carbon homes that address resident needs, and valuing and retaining that advice across the build programme. Developers would benefit from additional advice (a low carbon consultant) at an early stage for low carbon homes, prior to the planning application, unlike current low-rise housing developments that do not typically require input from M&E engineers. Retention of this low carbon advisor would ensure that design development and any value engineering continue to deliver the design intent and avoid costly additional works and reputational damage if the housing scheme does not perform well. The benefits of a low carbon advisor are further explored in Section 10.2.2;
- Developing the building fabric and building services to an equal RIBA stage prior to tendering a project should minimise design work on site, or reliance on contractors devising ways to install equipment when faced with issues. In an extreme case, this can result in the need to make a retrospective planning application (roof terminals at Active Homes), with programme delays;
- Embracing lean design, by ensuring the equipment and its performance is of an equal standard (not a mix of top-of-the-range and entry-level) and focusing on the quality of the installation, supporting site staff until they gain experience[footnote 60]. This is less about cost reduction and more about maximising the value of the available budget;
- Delivering to the planned programme, rather than incurring a significant over-run, will avoid unplanned cost increases arising from the cost of capital, additional site overhead costs, contractor claims, and potential purchasers dropping out. This further emphasises the need to ensure that low carbon expertise is introduced early and retained throughout the project, particularly for initial schemes.
Employing expert advice creates a cost challenge for those delivering smaller low carbon developments. The cost of MEP/low carbon advice per dwelling in a 400-home development will be very much lower than the cost per dwelling for a scheme of 15 homes. SME builders typically constructing smaller schemes could be provided with access to either free or part-funded advice for initial schemes as they gain experience.
Using MMC may be a means to increase supply, as it contributes to a fabric first approach, and can assist in addressing labour shortages through increased pre-fabrication. It was used at 3 of the 4 case studies. However, the potential benefit of a reduction in the on-site construction timetable due to the use of pre-fabricated panels, and the resultant reduction in overall costs, was not realised at these case studies.
Developers said the additional costs of delivering low carbon homes mean they will be outbid for land. Developing schemes without significant extra cost, or if the landowner or planning authority sets a requirement for low carbon homes, will overcome this barrier.
Increased levels of finance for new low carbon homes are available, such as the funding attracted by Project Etopia; investors noted this is becoming even more readily available. Recognition of the climate emergency has driven this, as investors want to move out of fossil fuels, in line with their Environmental, Social and Governance (ESG) strategies.
5. Counter to consumer perceptions, the case study homes were no more costly to buy than standard homes (based on the low carbon features), but the broader issue of insufficient low carbon homes and easy-to-access information about them remains problematic
Many potential customers perceive low carbon homes as costing more than traditional alternatives. However, an increased sales price was not observed across the case studies[footnote 61] as noted above, and the lack of availability of low carbon homes is a greater barrier in practice, at present.
While a sales cost increase was not applied, there was a capital cost uplift in each case, which the commercial developers chose to address in a variety of ways linked to business models. For the social housing developer, the capital cost uplift was met by a Welsh Government grant investing in innovation projects.
Accommodating the capital cost increase through a decrease in profit may not be a widely acceptable approach, even for pilot schemes. Instead, attention needs to be paid to ways to minimise or avoid an increased capital cost as discussed above, therefore avoiding the need to significantly increase the sales price.
In some cases, a sales cost uplift will be applied, possibly to reflect additional measures selected by a prospective purchaser, or as required by the LPA. Those who finance mortgages will require a better understanding of the benefits to residents in terms of lower expected running costs, notwithstanding the need to minimise the additional capital costs discussed above, so they will approve lending of the required additional funds.
6. There is likely to be an increased demand for low carbon homes if developers market them with details of features and running costs and provide post-occupancy support to ensure they perform as promised
Residents and potential residents (especially some demographic groups) saw a lower environmental impact from their home and/or using a renewable source of energy as a principal driver. It supported their personal values (see Section 6.6), albeit they also expect lower energy bills as a consequence.
Developers need to market low carbon homes with details of the features and expected running costs and to provide support to residents. They must ensure good performance, across a range of metrics, is achieved in practice. Three of the case study schemes marketed their homes with respect to lower bills or low carbon. However, lower energy bills were not always delivered (see Sections 4 and 7.5).
Three case studies kept their previous EPC rating, and only one dropped down a band, when the research team calculated the SAP rating for a sample dwelling taking account of observed performance issues (see Section 7.3). Some developers are also using their own terms as an alternative to EPC ratings, such as ‘Energy Positive’ at Corby. More widely, potential consumers noted a lack of information about carbon performance, and some of the interviewed residents felt their homes were insufficiently tried and tested (see Section 6.4).
A means of distinguishing between different levels of low carbon home in the marketplace would enable clearer marketing of the benefits. This could be through extending the ratings of the Energy Performance Certificate (for example, introducing an A+ and an A++ rating). A standard definition widely accepted by industry, rather than each developer using its own definition, would provide certainty for buyers/renters and lenders. Any certification scheme would need a body to undertake and administer the certification.
When developing housing schemes, and to support confidence in the as-built performance, developers and design teams need to consider future residents from the outset. These prospective residents are likely to be wary of unproven technologies (as noted by feedback from the case study residents and the wider consumer group) and several questions remain: will they work, is anyone available to maintain them, how might they need to behave differently, will they be able to understand the control systems?
Support from the developer is needed from the point of initial engagement, through the sales process, to handover and beyond, to ensure the homes can deliver the intended performance and comfort at an affordable running and maintenance cost. At individual sites, specific handover programmes need to be developed to explain how systems work together, not simply how they work in isolation. This is also an opportunity to provide additional advice on energy saving measures and sustainable living practices. At Marmalade Lane, residents felt that the sharing ethos of the co-housing community assisted in optimising the energy performance and running costs of their homes.
Developers can also consider helping prospective buyers choose low carbon enhancements prior to purchase. If buyers are offered additional low carbon features compatible with the design, and if they are informed about the capital cost and expected savings in running costs and carbon, they can make an informed decision. Some residents at Marmalade Lane were more disappointed that they could not have PV panels installed by the contractor (as an option) and had to retrofit them instead once they had bought their homes. Another example could be battery storage. The additional cost will be typically less than if retrofitted[footnote 62] and added to a mortgage.
Residents need a system of redress if the performance of the homes falls significantly short of what they were led to expect when deciding which home to move in to.
7. The construction sector and the energy services sector need to better engage. This will support delivery of low carbon homes, while avoiding significant energy infrastructure costs
Energy infrastructure costs can contribute to capital costs of low carbon homes, yet the housing sector is largely unaware of developments in the energy sector. Understanding energy transition and what this means for energy use at a domestic and development level, and to minimise up-front investment costs, is essential.
While developers are being charged with delivering low carbon low cost housing, many of the potential solutions and innovations are arising from the energy sector – utilities, appliance and control manufacturers, and innovative business model / service providers. The exclusion of energy systems solutions from Building Regulations means they do not form a central part of the design process; their carbon reduction benefits are not recognised in the regulatory structure, and they are unknown to most developers. If they were recognised by The Regulations, this would create an incentive for collaboration and adoption.
The culture and skills in these 2 sectors are different and need to better align to work together and understand the solutions each can provide. This will influence capital cost optimisation and overcome the barrier of concerns around new technology, its cost, challenges in operation, and reliability. Residents should benefit from designers’ better understanding of the operation of systems and running costs, and other influencing factors.
The funding of energy infrastructure is a barrier for developers. Traditional investment in the regulated electricity and gas network infrastructure is heavily funded through ongoing network charges on energy bills for consumers, but this funding model will not apply to many energy infrastructure investments to deliver low carbon homes. The only mechanism developers have to recoup this cost is through sales prices. To overcome this, there may need to be new regulatory structures which support new forms of business model aimed at funding and promoting low carbon infrastructure. This would allow developers (and house buyers) to avoid up-front low carbon infrastructure costs, thus creating a ‘level playing-field’ with traditional developments. This may mean moving away from ownership models to consideration of integrated service models such as heat-as-a-service (for example,fixed monthly costs for equipment and energy, maintenance provision, no concerns about replacement costs), similar to Personal Contract Purchase (PCPs) for cars. The inclusion of one or more energy service providers or innovative solution providers as part of the Future Homes Hub task force would assist in bringing these 2 sectors together.
10.2.2 A discussion on how to ensure low energy and carbon performance
Delivering low carbon homes that perform well against a range of metrics is essential to support the demand for such homes, and to ensure the drivers and expectations of low carbon homes are delivered in practice.
Seven learning points have been identified:
-
Performance in use should be improved with the adoption of simpler designs, which are easier to build and commission, and easier for residents to operate in accordance with the design intent.
-
Industry should undertake further product development and housebuilders need to improve their knowledge of existing and emerging products and services.
-
Developers and design teams should adopt a range of output metrics, not only low carbon targets.
-
It is essential to ensure planned performance is delivered so that both running costs and carbon emissions are low.
-
Developers need to focus on tailoring and resourcing the handover process for low carbon homes, so residents understand and operate their homes in an efficient manner.
-
The interaction between dynamic energy use and the energy system should be better recognised within the Building Regulations.
-
There is a need to upskill and improve collaboration across the industry.
These points are discussed below.
1. Performance in use should be improved with the adoption of simpler designs, which are easier to build and commission, and easier for residents to operate in accordance with the design intent
Design teams and developers either need to employ simple design solutions that an occupant can manage, or they need to recognise that residents of homes with more complex designs are likely to require third party support. Homes intended to reflect the dynamic nature of the energy system, and potentially targeting a net zero carbon outcome, will be likely to require an energy services provider to manage the technologies on behalf of residents.
It is better to optimise the design, with a focus on passive design and enhanced fabric performance to reduce demand, than being overly reliant on complex renewable energy solutions to achieve low carbon.
The case studies show the challenges in both delivering complex solutions and residents learning to operate them (see Sections 6.5 and 7.2). The case study developers who prioritised passive design and enhanced fabric performance achieved better social, economic, and environmental outcomes.
Residents were more satisfied with homes that had simpler services designs. This reflects a combination of the more complex services strategies not working effectively, concerns from residents about whether these issues would be addressed, and the systems’ complexity of operation. More complex low carbon homes may not be well suited for some residents (those who are less technically minded) – systems need to be suitable for the range of users.
The 2 schemes that had the more complex building services strategies, should, in theory, have provided greater carbon reduction benefits. However, there did not appear to have been an in-depth study of the challenges to delivery and operation by the project teams, and the capability to meet these challenges accounting for knowledge of existing performance gaps. Ultimately, the schemes were shaped by their aspirations, supported by grant funding for innovation. Simpler design solutions are easier to deliver and operate. In the research team’s view, a simpler solution is likely to perform best – achieving low carbon and lower operational and maintenance costs, satisfying residents, and being lower cost to construct.
Third party expert management of systems may be an option to ensure that homes operate effectively with minimal resident involvement beyond setting their comfort requirements. This was initially proposed at Etopia Homes. A new business model could offer comfort as a service where residents hand over the control of their systems to an energy services company, who would automatically and efficiently operate them according to comfort levels agreed by the resident. However, simplicity of design would still be important in such an instance, to improve the services such companies could provide.
Case studies could have performed better with good early-stage advice and improved site practices. Problems arose either because there was a complex concept or, where there was a simpler design, because people did not have the knowledge and experience to get the detail right. Fundamentally, none of the case studies demonstrated sufficient central responsibility for the initial low carbon design concept through detailed design, construction, commissioning, and handover. Some teams wanted to take a lead but lacked the expertise, while others focused more on design aspects rather than taking a holistic view. There was no one overseeing the quality of workmanship, ensuring the design intent was understood and delivered throughout the process, or responding to queries as they arose.
The project identified a number of industry-wide challenges regarding demand and performance, see Table 8.
Table 8: Industry-wide challenges regarding the demand for and performance of low carbon, low cost homes
Challenges
1. Developers and designers need to deliver a comparable, or better, user experience for residents:
- Ensuring comparable, or lower, energy running costs than in traditional homes, bearing in mind the much higher unit cost of on-peak grid-supplied electricity compared to gas;
- Ensuring novel systems are not a deterrent for future occupiers and that they will be operable with minimal expertise or training. They are used to traditional homes heated by radiators controlled by a single programmer and TRVs, and often with instantaneous hot water;
- Ensuring residents understand when and how to operate the equipment for maximum efficiency, such as operating an ASHP in the daytime, at a better CoP and supplied by PV, rather than in early mornings and evenings;
- Avoiding constraints on resident behaviour - heating switching off when doors or windows are open - or as a minimum, residents should be aware of and able to over-ride controls (albeit with a possible energy penalty).
2. Designers need sufficient skills and experience:
- To take the full benefits of the reduction in heat loss and reduce the heating capacity of the services accordingly. Oversizing equipment (kW) may result in poorer efficiency for most of the time. Matching products must be available;
- To ensure there will be sufficient hot water. Heat pumps typically deliver hot water at lower temperatures (meaning fewer showers or baths for the same stored water) and recharge rates are slower than with boilers. Needing to use an on-peak electric immersion heater boost will increase running costs, potentially significantly;
- To allocate sufficient and suitable space for services and minimise the impact on performance (avoiding long duct runs, multiple bends), whilst also considering the weight of equipment and installation routes;
- To select the location of external equipment to minimise potential disturbance within dwellings (such as noise and vibration from ASHPs);
- To design systems in a way that means they are compatible and can be operated (ideally) from a single control system;
- To consider maintenance requirements and costs for residents (filter changes to MVHR units) and for tradespeople.
3. Developers and design teams need to look to the future:
- To consider the replacement of technologies at the end of their life, the costs are likely to be much higher with a traditional boiler solution. This could be a deterrent for house buyers and landlords;
- To consider future energy system transitions – the use of batteries, time-of-use tariffs, electric vehicle (EV) charging etc. A greater electrical capacity (dual-phase) can increase capital costs and impose constraints on contractor selection and maintenance providers.
2. Industry should undertake further product development and housebuilders need to improve their knowledge of existing and emerging products and services
There is still the need for some innovation/product development. This includes mainstreaming smaller ASHPs in the UK new build housing sector, integrated controls to enable residents to operate their low carbon technologies in combination, and further product development of the panelised construction solutions used in the case studies. Barriers include the lack of common standards (for example, for MMC, which led to problems with obtaining warranties in the case studies), and the time and cost required to test and certify products and for them to be included in SAP – although clearly it is important that this is done thoroughly.
To benefit from the carbon, cost, and time savings that MMC can deliver, there is a need to plan the programme and site logistics for MMC from the start of a project, to consider detailing (particularly at interfaces), and for training.
Some low carbon systems will necessarily be complex, for instance, communal heating. Sufficient expertise is critical to ensure successful design, installation, commissioning, and operation. At Marmalade Lane, a simple communal heating system was very challenging for the relatively inexperienced stakeholders to deliver and manage, while at Tallack Road the innovative, more complex communal heating system was delivered and managed by experienced stakeholders, with better success.
Some participants at our energy systems stakeholder workshop identified SAP as a barrier to innovation. While SAP is a compliance tool, not a design tool, designers often focus on what is more cost-effective to achieve compliance with SAP. Similarly, if a technology, or combination of technologies, is not easy to model in SAP, this acts as a deterrent to its adoption. SAP therefore has a large impact on the design strategy selected.
The research team agrees that tools used to determine compliance, like SAP, need to accommodate new technologies, making it simple to include these technologies and approaches in modelling to assess their contribution. Otherwise, an inability to model some options could provide a barrier to their inclusion or lead to inconsistent data inputs or workarounds by assessors to find solutions. Some innovative features from the case studies could not be applied in SAP or did not receive full low carbon benefit[footnote 63]. However, it is important to ensure that the SAP methodology is robust, which can impact on how the more innovative technologies are represented, and the process of applying for inclusion within SAP.
SAP 11 is intended to be a significant step-change to recognise the increased pace of decarbonisation within the building stock, account for new energy-saving technologies and construction techniques, and the potentially significant changes in how we use energy in homes. BEIS has commissioned a project team to deliver SAP 11, building on the recommendations from an earlier SAP 11 scoping study[footnote 64]. BEIS will engage with industry as the project progresses through the existing SAP Industry Forum. SAP 11 will come into force in 2025, alongside the Future Homes Standard update of the Building Regulations.
3. Developers and design teams should adopt a range of output metrics, not only low carbon targets
A range of issues beyond carbon should be considered to ensure best outcomes. These include running costs, comfort, usability, and minimising additional capital costs[footnote 65]. Considerations should be captured in targets set in the brief, otherwise there is a risk of unintended consequences – like higher energy bills, greater maintenance requirements, and overheating. Designing homes in this way will require new skills, sharing of experience, and a culture change, see Table 9.
In terms of running costs, there are ways in which the consumption of grid-based electricity (or other heating sources) and the cost of energy can be reduced, through on-site generation, energy storage, and use of lower tariff electricity. Any modelling used to support design decisions will need to accommodate this.
Table 9: Essential design considerations
Essential design considerations:
- Capital cost: Site, building level and energy infrastructure
- Build quality/performance gap: The performance gap still exists, and is more significant where there is a better performing building fabric
- Space heating: Selection (source and emitters), sizing, location, controls
- Hot water: Quantities/stored water volumes, recharge rates, top-up approaches, internal heat gains from stored hot water
- Ventilation systems: Selection, provision of secure ventilation, commissioning, controls, advice
- User understanding: Concept and individual elements
- Internal comfort: Winter and summer, and minimising overheating risks
- Handover: Process and detail
- Energy use and carbon emissions: Role of on-site generation and storage
- Running costs: Tariffs, energy bills and replacement equipment costs
4. It is essential to ensure planned performance is delivered so that both running costs and carbon emissions are low
Ensuring the delivery of planned performance relates to energy use, carbon emissions, running costs and comfort.
Case studies did not deliver lower than typical running costs for a number of reasons. At some schemes, there was a significant performance gap that led to increased running costs, and there were some overly complex building services designs. This meant some residents experienced bills significantly higher than they expected. The other key contributor to higher costs was the big difference in unit costs of gas vs electricity, which was not negated by improved efficiency of space heating and hot water generation.
It is important that residents have confidence in their ability to operate their homes to ensure they are comfortable and energy costs are affordable. Lower running costs were cited in Section 6 as a key driver for residents. Reducing fuel poverty is a key driver for some developers, especially social landlords. Some potential residents may pay more for a low carbon home on the expectation of lower running costs, further reducing the barrier of additional capital costs. If this primary driver is not delivered in practice, it will send negative signals to the market – both those seeking to deliver low carbon homes and those planning to live in them.
As detailed in Section 7, the failure to deliver expected running costs in cast study homes was due to:
- design changes – in some cases driven by a desire to reduce the additional capital cost;
- the performance gap – building fabric and services;
- systems that were complex to understand;
- the absence of the intended access to lower cost tariffs and export tariffs;
- an assumption that a low energy/low carbon home would, by default, have a low energy bill
The performance gap and complex systems: The findings indicate the need for a greater focus on achieving performance - both via the building envelope and via heating and ventilation systems that are easy to use. Simpler approaches will reduce reliance on construction quality and handover, though these remain fundamental too.
Every development needs a team member to champion good design throughout the design and construction process, who also considers resident use. The cost for expert low carbon support throughout the process is small compared to the technology cost involved and it is crucial for performance, particularly during the initial period as industry becomes familiar with new practices. One example is a Passivhaus advisor in tandem with Passivhaus certification – a system which provides quality assurance for the design and construction of low energy buildings. Advice costs can be reduced as developers and their contractors become familiar with new ways of working.
Insufficient design co-ordination prior to starting on site, and insufficient detailing, will result in workarounds, missing insulation, poor airtightness and so on. These issues need to be addressed at construction stage or they could cause long-term performance problems for the building.
There were shortcomings with respect to thermal bridging, missing insulation, inefficient routing, and inadequate commissioning. While these issues are by no means specific to low carbon homes, the impact on the dwelling’s performance is more significant when the targeted heat loss is lower and unit energy costs are higher. There is a need to highlight the importance of getting designs right, ensuring they are buildable, and adopting best practice on site. Increased on-site testing will also be required to identify issues. Improved commissioning skills will also be required, for example commissioning of the ventilation system to balance supply and extract, and to match household size.
The introduction of more post-construction tests and the use of thermal imaging, linked to Building Regulations compliance, would highlight the issue. This should be supported by training and guidance via the hub (see item (h) below).
Low running costs: Until recently, gas was a low carbon form of heating and was also considerably cheaper (p/kWh) than oil or on-peak electricity. However, with the decarbonisation of the grid to date, and further reductions planned, electricity is becoming the lowest carbon form of heating (excluding renewable energy) when used in conjunction with heat pumps (individual or communal). This means that moving to a lower carbon form of heating is no longer associated with lower running costs, rather it may well increase running costs. This would certainly be the case if the energy demand were not also reduced in the low carbon home.
The seasonal variation in performance of renewable energy-based systems, and greater variation in running costs across the year, may impact on residents’ ability to pay, especially those unable to access payment via equal monthly direct debits. This is because ASHPs do not perform as well at the lower external air temperatures in winter and PV panels will typically generate less electricity to offset the purchase of grid electricity.
5. Developers need to focus on tailoring and resourcing the handover process for low carbon homes, so residents understand and operate their homes in an efficient manner
When designing low carbon homes, it is vital that each developer team avoids ‘undertaking an experiment’ to determine their ideal solution in light of new regulations. Some residents reported that they felt they were ‘guinea pigs’ when living in their low carbon homes and that systems were insufficiently proven. This cannot be repeated on a larger scale. Considering the users from the outset and investing in appropriate guidance and handovers will assist. This is to ensure that the design intent is delivered in practice in relation to energy use, carbon emissions, running costs, and comfort for the occupant.
Section 6 showed that the case study residents felt the handover process was insufficient for the complexity of the installed systems, or that it was delivered at the wrong time (on moving-in day), or both. Handovers for some case studies were during the COVID-19 pandemic and so they were scaled back, but this will not have impacted on the material provided.
Some residents were told that technologies had been set up correctly and there was no need to adjust anything. This does not demonstrate a good understanding of systems on behalf of the sales team, and in some cases the commissioning was not correct, and adjustments were required. It is quite reasonable for residents to want to understand their heating, hot water, and ventilation systems and how to control them to ensure comfort, manage their energy bills, and adapt to changing circumstances. The handover process needs to be properly resourced so guides, rather than manuals, explain how systems work in an interactive manner. Users expect an integrated control system, not separate controls for the space heating and ventilation systems.
6. The interaction between dynamic energy use and the energy system should be better recognised in the Building Regulations
It is necessary to account for changes happening to the system to optimise energy use, carbon, and running costs. These changes are currently not fully accounted for in Part L and SAP. These can be characterised as long-term grid decarbonisation, and short-term fluctuations in grid CO₂ intensity and wholesale price.
Long-term grid decarbonisation: Most new homes are expected to use a heat pump. As the grid decarbonises, CO₂ will become a less important measure of the energy performance and energy efficiency of the heating system as the energy supply is on a trajectory to zero carbon emissions. Part L 2021 and SAP have looked to address these changes in 2 ways.
- The carbon emission factors in SAP 10.2 have been updated to reflect grid decarbonisation;
- The introduction of a primary energy target metric in Part L in England establishes a separate target for energy use.
This report endorses the review of metrics recommended in the SAP 11 scoping study[footnote 66]. The focus should be whether the primary energy target is the best metric or whether other metrics, such as space heating demand, better reflect the performance of the home, as primary energy also accounts for the efficiency of the energy supply system.
There is also a need to consider the potential increase in energy costs in moving to a low carbon heating system. The unit cost of electricity was 4 to 5 times higher than gas during this research project[footnote 67]. Moving to a lower carbon and more efficient heat pump will likely result in higher fuel bills than a gas boiler. This increases the financial benefit of improving the fabric efficiency of homes, as well as taking advantage of time-of-use tariffs.
It was also noted that the government is reviewing the way social costs are applied to different fuels as noted in the Net Zero Strategy, and this may reduce the relative difference in unit costs between fuel types.
Short-term dynamic carbon intensity: Exploiting the short-term dynamic CO₂ intensity and pricing could help lower running costs and carbon emissions from new housing. There are potential benefits arising from energy storage on-site, such as through thermal or battery storage, including the use of electric vehicles.
Energy storage allows the occupant to maximise the use of energy generated on-site, and, with the adoption of time-of-use tariffs, allows residents to import energy from the grid during periods of lower tariffs (and potentially lower real-time CO₂ impacts) and to use stored energy during periods of higher tariffs (when the grid is higher CO₂ intensity). This could contribute to reduced running costs for residents, benefits that could part-fund the role of a third-party energy company. It may also benefit local network capacity through reductions in peak loads, and national generation capacity.
The inclusion of short-term dynamics in Building Regulations and SAP could help to drive optimised design and performance. This would encourage developers to explore emerging solutions. SAP would need to be more dynamic to capture such benefits, reflecting dynamic costs and CO₂ intensity, to correctly assess cost and CO₂ impacts, alongside being able to represent a much broader range of low carbon solutions and services (for example using a time-of-use tariff, or optimising different technologies as part of a complete system).
It was noted that the SAP 11 scoping study[footnote 68] recommends the inclusion of an output related to peak demand and/or demand management, which would allow SAP to value strategies aimed at reducing peak demand and at shifting demand for system flexibility. The SAP 11 project team is considering the recommendations from this scoping project.
This needs careful consideration. Any change to SAP must account for the inherent unpredictability of dynamic carbon intensities, which are highly variable in terms of both time and location. Furthermore, this variation will be influenced by the grid decarbonisation pathway. It is important to ensure that the changes are tested so that they drive improvements in performance, free of negative consequences.
7. There is a need to upskill and improve collaboration across the industry
There is a broad need to increase low carbon skills. This was evidenced in the social research and by site observations, which highlighted insufficient knowledge and skills across the industry.
Complementary to this, there is benefit in specialist advice. This avoids each team ‘reinventing the wheel’ as they start to design and deliver low carbon homes. Support could be either via an expert hub, engaging a low carbon advisor, or both.
The requirement for additional effort and support will likely reduce after 1 or 2 developments have been delivered. However, new products and services are likely to continue to be developed which will deliver further performance improvements and would benefit from continued specialist advice.
Benefits of a low carbon advisor: The case study observations showed that most of the problems on site stemmed from design – lack of co-ordination and poor design advice – rather than poor workmanship. This was confirmed by interviews with the trades people who did not identify a lack of construction site skills as a barrier. The first time a new activity is encountered, it is likely to take a few days longer, but people typically soon acquire the required skills.
The quality of design advice matters. Projects would benefit from the appointment of a low carbon advisor from the concept design stage through to delivery and handover. A low carbon advisor would be an informed member throughout the project, contributing their expert knowledge, and representing future residents. Their engagement across the RIBA Plan of Work[footnote 69] stages will ensure that the early vision is conveyed throughout, a balance is struck to optimise the design, the performance gap is addressed, and unintended consequences from value engineering and later-stage decisions are avoided. This person should also guide the handover process.
Upskilling across the industry: Better knowledge, application, and collaboration is needed across the entire construction sector to improve the design, delivery, sales process, and in-use performance of low carbon homes. These are discussed below.
Developers and housebuilders need to embrace the diverse range of drivers. They need to recognise and value specialist advice provided at an early stage (which was understood at Tallack Road). In addition, the planning professionals providing early feedback (at the pre-application stage) need to understand applicants’ aims and the impact that planning decisions can have on the delivery of these aims (cost and performance).
Design team members will also need new expertise - those developing schemes and those costing them. To ensure effective design and delivery of low cost, low carbon homes, designers will require a better understanding of the merits of a highly insulated, airtight fabric designed and detailed to avoid thermal bridges, as well as of the common issues that prevent this being delivered in practice. This entails a better understanding of the integration between low carbon technologies and the building fabric, and the opportunity to optimise designs for use within a more flexible energy system, such as using energy storage.
Contractors need to value, and draw upon, those with suitable skills, those with good knowledge who are happy to impart it to others. They need to understand the potential impacts of standard on-site practices on the performance of low carbon homes and running costs, and why new approaches and skills are required. This will reduce tender costs through better efficiencies and avoid premiums for unknown items. This sub-sector also needs to understand future changes, set out in a roadmap, to be confident of the benefits of upskilling.
New on-site skills will need to be learned about how to accommodate designs with greater thicknesses of insulation than perhaps workers are familiar with, and the importance of reducing thermal bridges in buildings with a high-performance building fabric. A lack of attention to detail can manifest itself as a significant deterioration in performance when high performance standards are targeted. Other site-based skills will be needed in respect of the installation and commissioning of equipment, such as heat pumps and MVHR. Enhanced pre-handover testing may be required. Manufacturers may choose to provide training, aware there may be a tendency for sub-contractors to favour their equipment.
The Site Manager and Sales Team need to ensure the homes are delivered well and properly handed over. This requires clear responsibility and adequate resources for the task.
A further key issue is the repair and servicing of the technologies. Those responsible for property maintenance and repair will need new skills to respond to call-outs and planned maintenance of new technologies. Difficulties finding someone to fix things if they go wrong, and maintenance of technologies, were the main perceived challenges to living in a sustainable home identified by respondents to the wider consumer survey (picked by 39% of respondents). This was not something explored in-depth across the case study homes as they were new and performance issues tended to be associated with installation, and the responsibility of the developer, rather than maintenance issues.
There is a need for monitoring the performance of low carbon homes to ensure the design intent is followed through, or to identify under-performance. This is a specialist skill set developers will need to value and fund. Such skills are not widespread at present.
The final group who needs additional knowledge is the general public. This is to stimulate interest in low carbon homes and to ensure that they have a correct understanding and avoid any misconceptions. This could be delivered via the hub as below.
Sharing of information and provision of support via a hub:
A hub to share knowledge and guidance should help to deliver improved energy and carbon performance. The target audience is the wide range of stakeholders contributing to the delivery of low carbon homes. A central aim is to help overcome the barrier for SMEs who build small developments and for whom the cost of advice per dwelling may be prohibitive. The hub should also bring together the construction and energy services sectors to discuss and address the role of housing in the future energy system.
The research team notes that the Future Homes Hub has been established, which may be looking to deliver this remit, but which is mainly focused on ways to meet the Future Homes Standard (FHS). We see a need for a hub focused more broadly than the FHS, considering how to reduce embodied carbon and close the performance gap. Other bodies are also active in this area – the UK Green Building Council (UKGBC), the Good Homes Alliance (GHA), the Passivhaus Trust, and the AECB are examples, but a unified approach may be more beneficial.
10.3 Conclusion
BEIS commissioned the Building for 2050 research project to understand the drivers and barriers to large-scale construction and take-up of low cost, low carbon housing. The research team was tasked with identifying key lessons to minimise cost premiums, improve energy efficiency, reduce carbon emissions, increase consumer demand and accelerate industry delivery.
The aims have been achieved through investigating new low carbon housing from inception to occupancy. The unique research combined physical and social research with on-site observations and in-use performance monitoring to deliver a detailed evaluation of 4 low carbon new build housing schemes. These findings have been complemented by wider perspectives from the development and construction sector, the energy industry, and consumers.
Ultimately, the report sets out the key steps to achieve the wide-scale construction and take up of low cost, low carbon homes which deliver low energy and low carbon performance. These homes should be net zero ready, with no need for major retrofit before 2050.
-
The report defines low cost, low carbon homes as low carbon homes with a lifetime cost similar to, or lower than, standard new homes currently on the market. In terms of emissions, low carbon homes in this report are equivalent to Code for Sustainable Homes 5 or 6 (or Code 4 with a fabric first approach) or Passivhaus. ↩
-
Heat and Buildings Strategy, HM Government (2021), p.29. ↩
-
The Code for Sustainable Homes was an environmental assessment method for rating and certifying the performance of new homes. In some cases, meeting particular levels of the code was a requirement under local authority plans and social housing funding. In such cases, the energy requirements were typically better than the Part L minimum standards. ↩
-
The Climate Change Act 2008 (2050 Target Amendment) Order 2019 ↩
-
The Net Zero Strategy: Build Back Greener, HM Government (2021) ↩
-
Part L 2021, DLUHC (2021) ↩
-
Approved Document L: conservation of fuel and power, Welsh Government (2016) ↩
-
Building Regulations - energy standards and associated topics - proposed changes: consultation, Scottish Government (2021) ↩
-
Energy Strategy for Northern Ireland Consultation on Policy Options, Department for the Economy (2021) ↩
-
The Net Zero Strategy: Build Back Greener, HM Government (2021), p.141; Heat and Buildings Strategy, HM Government (2021), p.11. ↩
-
The carbon emissions arising from energy used by fixed building services, as defined in Approved Document Part L of the Building Regulations. ↩
-
The CCC stated in its report Net Zero: The UK’s contribution to stopping global warming, CCC (2019) that achieving the net zero target will require the full decarbonisation of buildings by 2050. ↩
-
Delay was due to complications with the heating system and the COVID-19 pandemic ↩
-
Monitoring continued beyond the Building for 2050 research in line with the requirements of the grant funding. It has been reported to the research team that most of the flats/homes are performing as intended. A small number of homes have higher energy use, which is believed to be due to problems with the batteries and/or PV systems. ↩
-
The Building for 2050 research was focused on the first 10 homes. The later homes used ASHPs or electric panels for space heating in the houses and apartments respectively, rather than the combined GSHP/EEB/PV-T system. ↩
-
More panel types have since been introduced, and new schemes have been designed by the developer to use the system from the outset ↩
-
The London Plan, March 2016, consolidated with alterations since 2011 ↩
-
This is likely to be because of the COVID-19 pandemic. ↩
-
The service charges did include part of the energy costs – for the operation of the communal system and its maintenance. ↩
-
The developer had intended to offer residents a personal explanation of how to operate the low carbon technology in their new homes, but this did not happen because of the COVID-19 pandemic. ↩
-
It was not possible to heat other rooms without also heating the living room. ↩
-
For example, Heffernan, E., Pan, W., Liang, X., de Wilde, P., Zero carbon homes: Perceptions from the UK construction industry. Energy Policy 79, 23–36 (2015); Joseph Rowntree Foundation, Building sustainable homes (2015); Zero Carbon Hub, Closing the Gap Between Design and As-Built Performance: End of Term Report (2014); UKGBC, Net Zero Whole Life Carbon Roadmap for the Built Environment (2021). ↩
-
DNO = Distribution Network Operator, the company that owns and operates the power lines and infrastructure that connect the network to a property. ↩
-
UKPN = UK Power Networks is a DNO covering Southeast England, the East of England and London. ↩
-
NHBC Foundation, 2012. Today’s attitudes to low and zero carbon homes: views of occupiers, house builders and housing associations: primary research. NF 40ii. Published by IHS BRE Press on behalf of the NHBC Foundation, Bracknell. ↩
-
NHBC Foundation, Savills, 2018. Beyond location, location, location: priorities of new-home buyers. ↩
-
This group included those who live in, have lived in, or have visited such homes. ↩
-
The individual batteries were Tesla batteries, hence the reference to the Tesla app. ↩
-
SAP 10 states that a unit of electricity generates 35% less CO₂ emissions than gas. ↩
-
Residents were delayed moving into the Active Homes scheme due to various site delays and the impact of the COVID-19 pandemic. This resulted in only having up to 4 months heating data available for this site. ↩
-
Units were selected from each scheme’s monitored houses and flats, to represent the median. ↩
-
The original heaters used were typical of those used in commercial installations, which rely on a minimum air flow of 2 m/s to prevent the element from overheating and cutting out, whereas domestic scale models can operate with a lower minimum air flow of 0.5 m/s – if this type had been used then air flow balance may have been achieved with the original strategy. ↩
-
The Building for 2050 research was focused on the first 10 homes. The later homes used ASHPs or electric panels for space heating and ASHPs for DHW in the houses and apartments respectively, rather than the combined GSHP/EEB/PV-T system. ↩
-
BRE Technical Note No: 1 – V1.0: Recognition of the GDC Group Ltd - Zeroth Energy System within SAP 2009 and 2012 assessments, BRE (2019) ↩
-
We were unable to do this analysis based on measured data as, apart from Marmalade Lane, we did not have data spanning a full year. ↩
-
This relaxation was introduced is to provide some relief for those who have to use more carbon intensive fuels, either because gas is not available or is not preferred because, for example, electrically driven heating, such as heat pumps, is preferred. This relaxation has been removed for Part L 2021. In particular, grid electricity now has a lower carbon emission factor than gas and therefore it no longer needs a fuel factor to support its use. ↩
-
Regulated energy includes space heating, domestic hot water, ventilation, lighting, and cooling, but excludes cooking and use of plug-in equipment. ↩
-
To minimise the incidence of respiratory infections and allergies, the RH range inside a home should ideally be between 40% and 60% (A V Arundel, E M Sterling, J H Biggin, and T D Sterling (1986) Indirect health effects of relative humidity in indoor environments). ↩
-
This reference is to the case study in Summer 2021. Dramatic rises in unit costs have been observed during 2022 and are predicted for 2023. The ratio of electricity to gas unit costs may therefore change from that observed during the research project. Higher price rises may be incurred by systems subject to commercial tariffs which are not covered by Ofgem’s domestic energy price cap. ↩
-
It was not the aim of the project to monitor the performance of individual low carbon technologies. ↩
-
Note that unit floor areas differ, as noted in the x-axis, and comparisons made should account for this. ↩
-
This factor refers to the case in Summer 2021. Dramatic rises in unit costs have been observed during 2022 and are predicted for 2023. The ratio of electricity to gas unit costs may therefore change from that observed during the research project. Higher price rises may be incurred by systems subject to commercial tariffs which are not covered by Ofgem’s domestic energy price cap. ↩
-
The dual phase arrangement is unusual and comprises a 3-phase supply to the dwelling, and 2 of the phases used for connection to different circuits. All equipment is single phase. Smart meters, required to enable access to a time-of-use tariff, were not available for a 3-phase supply. ↩
-
If the plans for a zero-carbon grid materialise by 2035 there will not be a material diurnal or seasonal variation in CO₂ intensity. However, with an increasing intermittent (and predominantly unforecastable) generation mix, price fluctuations are going to be large unless very large amounts of storage and flexibility services are delivered. ↩
-
Thermal storage is likely to make use of the domestic hot water cylinder, rather than needing an additional item of plant, but other options are available. ↩
-
The dual phase arrangement is unusual, and it comprises a 3-phase supply to the dwelling, and 2 of the phases being used for connection to different circuits. All equipment is single phase. ↩
-
This might also have exposed residents to the wholesale electricity market and taken away domestic consumer protection. This is an issue which needs to be considered in the round. ↩
-
News story: Government takes historic step towards net-zero with end of sale of new petrol and diesel cars by 2030, DfT (2020) ↩
-
PAS 1878 provides a technical framework for domestic Demand Side Response (DSR). It specifies the requirements and criteria that an electrical appliance needs to meet to perform and be classified as an energy smart appliance (ESA). It defines the attributes, functionalities and performance criteria for an ESA, and specifies how compliance with these can be verified. It includes both heat pumps and EVs. ↩
-
The report was first drafted in Autumn 2021 when this observation was made. ↩
-
Driven by the variable mix of sources, including wind power contributions, which are both seasonal and weather dependent. ↩
-
The predicted consumption was generated via SAP, which can overpredict demand for smaller household sizes as it reflects the property size. ↩
-
This factor refers to the case in Summer 2021. Dramatic rises in unit costs have been observed during 2022 and are predicted for 2023. The ratio of electricity to gas unit costs may therefore change from that observed during the research project. Higher price rises may be incurred by systems subject to commercial tariffs which are not covered by Ofgem’s domestic energy price cap. ↩
-
Officers may choose to outsource the expertise, rather than having it in-house. ↩
-
Developers may appoint a private sector organisation to this role. ↩
-
BS 40101 - Performance of occupied and operational buildings, BSI (2022) ↩
-
These are low carbon homes, not zero carbon homes ↩
-
At one case study a long-term relationship with a sub-contractor was helpful in the context of its first experience of installing an innovative heating system, rather than adopting a strictly contractual approach. ↩
-
The dwellings at Etopia Homes were more expensive than others with the same number of bedrooms in the local area, but this was influenced by the considerably larger floor area. ↩
-
Some items, such as battery storage, may come down in cost in the future with greater market uptake, but electrical works would also be required to facilitate a retrofit. ↩
-
This related to SAP 2012 and some of the issues were addressed in SAP 10.2. ↩
-
Making SAP and RdSAP 11 fit for Net Zero, Etude, CIBSE, Levitt Bernstein, Elementa, WSP, Clarion Housing Group & UCL (2021) ↩
-
Or to adopt business models that mean these costs can be recouped over a number of years, rather than in the sales price. ↩
-
Making SAP and RdSAP 11 fit for Net Zero, Etude, CIBSE, Levitt Bernstein, Elementa, WSP, Clarion Housing Group & UCL (2021) ↩
-
This factor refers to the case in Summer 2021. Dramatic rises in unit costs have been observed during 2022 and are predicted for 2023. The ratio of electricity to gas unit costs may therefore change from that observed during the research project. Higher price rises may be incurred by systems subject to commercial tariffs which are not covered by Ofgem’s domestic energy price cap. ↩
-
Making SAP and RdSAP 11 fit for Net Zero, Etude, CIBSE, Levitt Bernstein, Elementa, WSP, Clarion Housing Group & UCL (2021) ↩
-
The RIBA Plan of Work organises the process of briefing, designing, constructing and operating building projects into 8 stages and explains the stage outcomes, core tasks and information exchanges required at each stage. ↩