UK Net Zero Research and Innovation Framework
Updated 30 March 2023
Foreword
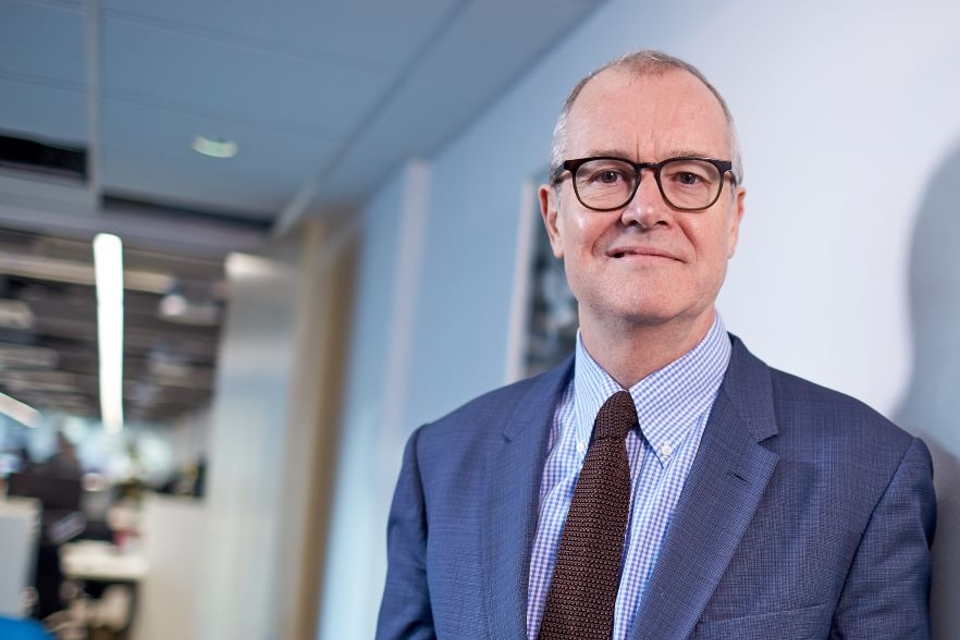
The UK has made real progress on delivering against our long-term greenhouse gas emissions reduction targets. Between 1990 and 2019, we grew our economy by 78% and cut our emissions by 44%, decarbonising faster than any other G7 country. However, achieving net zero will require profound changes across society and the economy. Greenhouse gases are emitted from every part of the UK economy and sustained, co-ordinated action across government, business, academia and civil society is required to meet our 2050 target. This transition will involve complex interactions between technology, infrastructure, people, data, institutions, policy and the natural environment.
As the Government Chief Scientific Adviser and National Technology Advisor, I will lead the newly established Office for Science and Technology Strategy (OSTS) to help the government use science and technology to address the most significant challenges of today and the future, including net zero. Net zero is a whole-system challenge - if tackled in the right way, it could create opportunities for increased prosperity, better social outcomes and a thriving natural world. A systems approach can provide the framework to lead change across both the public and private sectors, and the UK has world-leading capabilities in research and innovation to help inform the approach we might take. This will be supported by the new National Science and Technology Council (NSTC), chaired by the Prime Minister, which will provide direction on the use of science and technology to achieve strategic advantage for the UK.
Research and innovation will be an essential part of the drive to decarbonise. Success will require us to pursue both mature technology implementation and enhancement, together with more rapid pull through of emergent technologies and discovery and invention of new technologies for the longer-term. We can achieve this through a bold, coherent programme of public sector research and innovation investment alongside appropriate policy support, coordinated with industry, to encourage and de-risk technology development and deployment and mobilise private sector investment.
Produced under the guidance of the government’s Net Zero Innovation Board, this Net Zero Research and Innovation Framework represents a first statement of the UK’s net zero research and innovation priority areas over the next 5 to 10 years. It supports delivery of the UK’s Net Zero Strategy and carbon budget commitments, drawing on the existing evidence-base and research and innovation work already being undertaken. It identifies the main sectors and their respective challenges for the UK to reach net zero, the key research and innovation needs that should be addressed and the timescales for doing so. Government funded research and innovation, appropriate policy and regulatory support, private sector innovation, investor funding and academic research will all play a key role in delivering these.
This Framework will help to ensure that the UK’s public sector net zero research and innovation spending is aligned to agreed UK priorities. It aims to provide a clear signal to the private sector and our academic and research communities about the UK’s focus areas as we move towards 2050 and to lay the foundations for a collaborative, whole-systems approach to net zero research and innovation activity. Taken together with the Net Zero Strategy, this provides an initial roadmap for maximising the contribution of innovation towards net zero, understanding the social and economic drivers of change and supporting international science and technology collaboration. It will need to be quickly developed into a detailed plan for delivery against which we can track our progress.
Sir Patrick Vallance
Government Chief Scientific Adviser
UK Net Zero Research & Innovation Challenges
This Net Zero Research and Innovation Framework outlines the research and innovation required to support delivery of the UK’s Net Zero Strategy. A wide-ranging portfolio will be needed and this Framework details research and innovation challenges across the Carbon Budget and related sectors[footnote 1]:
- Power
- Industry and low-carbon hydrogen supply
- Carbon Capture Utilisation and Storage (CCUS) and Greenhouse Gas Removal (GGR)
- Heat and buildings
- Transport
- Natural resources, waste and F-gases
It also identifies cross-cutting and systems-wide issues and linkages between sectors extending beyond technology to include research and innovation related to systems, processes, business models and the socio-economic and behavioural considerations needed to encourage green choices. Ultimately, both investments to push forward technology development and policy mechanisms to create the conditions to pull innovations to market will be needed to accelerate pathways to net zero.
Figure 1: Key areas for research and innovation to 2050
Power
- Improving system integration, flexible demand and energy storage (2020s)
- Continual development of offshore wind (including floating) and earlier stage renewables (2020s)
- Developing nuclear SMRs and AMRs (2020s & 2030s)
- Utilising bioenergy and BECCS (2020s & 2030s)
Industry and low carbon hydrogen supply
- Improving resource and energy efficiency in industrial applications (2020s)
- Switching to low and zero-carbon fuels and feedstocks (2020s, 2030s & 2040s)
- Capturing and storing industrial emissions (2020s & 2030s)
- Efficient production of low carbon hydrogen at scale (2020s & 2030s)
- Bulk hydrogen transportation and storage (2020s & 2030s)
Carbon Capture Utilisation and Storage (CCUS) and Greenhouse Gas Removals (GGR)
- Capturing emissions efficiently and at low-cost (2020s & 2030s)
- Supply chain innovation for CCUS (2020s)
- Developing and improving transportation and storage of CO2 (2020s & 2030s)
- Developing and demonstrating GGR technologies (2020s, 2030s & 2040s)
Heat and buildings
- Building retrofit and energy efficiency solutions (2020s)
- Understanding feasibility and safety of hydrogen for heating (2020s)
- Further innovation of heat pumps, including installation and use (2020s)
- Researching and trialling heat networks and non-traditional heat sources (2020s & 2030s)
Transport
- Transport and mobility as a system (2020s)
- Decarbonising roads (2020s)
- Decarbonising railways (2020s)
- Decarbonising aviation (2020s, 2030s & 2040s)
- Decarbonising maritime sectors (2020s & 2030s)
Natural resources, Waste and F-gases
- Integrated and dynamic approach to land-use (2020s)
- Sustainably managing forests, peatlands and the marine environment (2020s & 2030s)
- Sustainable food and biomass production (2020s, 2030s & 2040s)
- Reducing and minimising waste and F-gases (2020s)
Whole systems approach
- Understanding optimal net zero pathways (2020s, 2030s & 2040s)
- Creating an integrated energy system (2020s, 2030s & 2040s)
- Enabling integrated, multi-modal transport systems (2020s, 2030s & 2040s)
- Developing digital solutions and unlocking resource and energy efficiency (2020s, 2030s & 2040s)
- Researching, understanding, and unlocking sustainable behaviours, business and financial models (2020s, 2030s & 2040s)
In the Power sector (Chapter 4.1), renewable energy generation will need to be rapidly deployed with research and innovation driving continual improvements and unlocking new opportunities, such as floating offshore wind, as well as enabling reliable low carbon electricity through nuclear. The wider electricity system must also transform to integrate large-scale and long-term energy storage and maximise the opportunities for matching flexible supply and demand. Innovation is also needed in biomass production, which has potential to aid decarbonisation across several sectors and drive negative emissions through carbon capture and storage.
In the Industry and low carbon Hydrogen Supply sectors (Chapter 4.2), continuing innovation to drive resource and energy efficiency alongside proving the feasibility and reducing the cost of low and zero-carbon fuels and feedstocks (such as electricity, hydrogen and biomass) will be critical. Residual emissions will need to be captured at source or offset (see chapter 4.3). In addition, hydrogen is expected to be a key energy vector with uses in industry, for heating, for low-carbon fuels and to support flexibility in the energy system. Research and innovation which supports scaling-up the supply and demand for low carbon hydrogen will be required through the 2020s-30s, including developing and demonstrating cost effective production, distribution and storage of low carbon hydrogen at scale.
For Carbon Capture, Utilisation and Storage (CCUS) and Greenhouse Gas Removals (GGRs) (Chapter 4.3), even in ambitious decarbonisation scenarios there are likely to be some residual greenhouse gas (GHG) emissions across the UK in 2050. This means research and innovation is needed to support deployment of industrial-scale CCUS technologies over the 2020s and 2030s, to develop other greenhouse gas removal solutions such as Direct Air Capture (DAC), for the deployment of CO2 transport and storage infrastructure, and for using captured CO2, for example, in the production of synthetic fuels.
In the Heat and buildings sector (Chapter 4.4), a key challenge is retrofitting the significant proportion of homes and non-residential buildings that require remedial work to improve efficiency and supply low carbon heat. Innovation in technologies, processes and business models is required to support this as well as research to understand how consumer behaviour affects uptake. Research will also support decisions in the 2020s on the most suitable options for heating our homes, including low carbon hydrogen and heat pumps, as well as the longer term role for heat networks.
In Transport (Chapter 4.5), electrification is expected to be the principal decarbonisation solution for passenger transport. However, other low carbon fuels, particularly low carbon hydrogen, may have an important role to play, for example in heavy goods vehicles, some buses and railways. Research and innovation is also required on refuelling and recharging infrastructure and how to integrate electric vehicles with the wider electricity system. Innovation is critical in aviation and maritime, which are amongst the most difficult to decarbonise sectors. Research is also needed to understand how we can make lives better by changing the journeys people make, bringing behavioural and technological solutions together at scale with an understanding of how we will travel within our communities.
For Natural resources, waste and F-gases (Chapter 4.6), there are multiple demands on land that influence how it is used. Research is needed to understand trade-offs and synergies between food production, forestry and biomass production, habitat and peatland restoration, biodiversity and urban expansion. Research and innovation is needed for methods of sustainably managing forests, peatland and the marine environment to promote carbon sequestration alongside wider environmental benefits; for scaling-up a sustainable bioeconomy, on the resilient supply and demand of agricultural products and promoting sustainable choices; as well as for tackling methane from waste and to develop sustainable alternatives to F-gases.
Underpinning all of this is the need for a whole systems approach (Chapter 3). This includes research to understand the interrelated nature of different sectors and between new technologies, consumer behaviour and business models. Cross-cutting themes and system of systems questions include understanding the optimum use of scarce resources; integration of digital solutions; and the need for broad public support of new technologies as well as the development of viable markets, regulatory arrangements and supply chains.
This Framework supports an integrated approach to net zero research and innovation planning within government (Chapter 5) and aims to provide businesses and the research community with a tool for their own research and innovation agendas. We intend to publish a follow-up detailed Delivery Plan to show which aspects of this Framework government investment is prioritising based on 3 factors: its impact on decarbonisation, potential economic opportunities for the UK and keeping open credible pathways to net zero.
1. Innovating for net zero
Innovation to enable net zero
In 2019 the UK became the first major economy to legislate to reduce greenhouse gas emissions to net zero by 2050. The Net Zero Strategy sets out the UK’s current pathways to net zero and to delivering Carbon Budget 6, which requires greenhouse gas emissions to reduce by 78% from 1990 levels by 2035. Achieving these goals will require strong coordination across the UK, the accelerated development and deployment of green technologies and increased energy and resource efficiency, the creation of new industries and business models, supportive policy and regulatory interventions and encouraging a shift to more sustainable green choices.
Research and innovation including investment in skills, infrastructure, technologies, knowledge generation and sharing, and stakeholder engagement will play an essential role in making this happen. Innovation and related policy support have helped the UK to decarbonise faster than any other major economy over the past 2 decades. The success of the offshore wind sector in the UK demonstrates what can be achieved through partnership between government and industry and by combining technology innovation with targeted incentives and policies for market deployment. As outlined in the Net Zero Strategy, research and innovation will further enable many of the changes needed to continue cutting emissions whilst maximising jobs, export opportunities and environmental benefits.
However, the scale of transformation is profound. Whatever the ultimate route, net zero will involve some combination of the following:
- Transitioning away from fossil fuels, with continued decarbonisation of our energy system, including electricity supply, heating and transport as well as growth in other low carbon fuels such as hydrogen, alongside large-scale and long-term storage to achieve increased system flexibility;
- Improving energy and resource efficiency across the economy, including moving to a circular economy approach ‘reduce, reuse, repair, recycle’, to reduce increasing demand for energy and carbon-intensive resources whilst delivering wider environmental benefits;
- Greenhouse gas removals at scale, through natural methods (such as tree planting) and through engineered technologies (such as direct air carbon capture and storage). Carbon Capture and Storage technologies are an urgent and essential component for all realistic pathways to net zero;
- Changes in land use to support carbon sequestration and clean energy, alongside sustainable food production, thriving biodiversity and climate change adaptation;
- Green choices and practices, including supporting people and businesses to use lower carbon products and services wherever possible.
This transition will involve complex interactions between technology, infrastructure, people, data, institutions, policy, and the natural environment. By taking a ‘systems approach’ government can help to navigate this complexity. Research and innovation will be needed in the technological, socio-economic and environmental spheres recognising that changes to one area can directly or indirectly impact others. Both investments to push forward technology development and supportive policy mechanisms to create the necessary conditions to pull innovation to market will be needed, engaging with both current providers of products and services and new entrants. It will be important to properly engage with end-users to ensure effective product and service design and to remain alert to any unintended consequences from introducing new technologies.
Benefits of innovation
Research and innovation can significantly reduce the cost of the net zero transition, nurture the development of better products and new business models, and remove barriers to adoption. Innovation has been key to many of the carbon savings seen to date and the cost of renewable energy technologies continues to fall (see examples in Figure 2). However, technologies needed to deliver almost half of the CO2 reductions required to reach net zero by 2050 are still in prototype phases[footnote 2] and will require continued investment in research and innovation to pilot, scale-up and commercialise cost-effective solutions. Estimates suggest that public R&D investment in key technologies could deliver between £54bn and £115bn of cumulative UK energy systems savings from 2019 to 2050[footnote 3].
Figure 2: Global Levelised Cost of Electricity (LCOE) from newly commissioned utility-scale renewable power generation technologies, 2010 to 2020
Source: IRENA (2021) Renewable Power Generation Costs in 2020, International Renewable energy Agency, Abu Dhabi. ISBN 978-92-9260-348-9
Note: This data is for year of commissioning. The thick lines are the global weighted-average LCOE value derived from the individual plants commissioned in each year. The project-level LCOE is calculated with a real weighted average cost of capital (WACC) of 7.5% for OECD countries and China in 2010, declining to 5% in 2020; and 10% in 2010 for the rest of the world, declining to 7.5% in 2020. The single band represents the fossil fuel-fired power generation cost range, while the bands for each technology year represent the 5th and 95th percentile bands for renewable projects.
Description of figure 1
Bar chart of change in levelised cost of electricity (unit used is the 2020 USD/kWh) for different renewable power generation technologies between 2010 and 2020.
- Biomass: no change
- Geothermal: +0.022
- Hydro: +0.006
- Solar Photovoltaic: -0.324
- Concentrating solar power: -0.232
- Offshore wind: -0.078
- Onshore wind: -0.05
Working back from 2050, major research and innovation challenges must be tackled this decade to keep the UK on track for achieving net zero and to remain globally competitive. Fast tracking carbon reduction requires gaining acceptance for and deploying at scale those technologies that are ready; accelerating the development of those that are not; and researching solutions to problems that do not yet have solutions. Appropriate policy support will help near-to-market innovations drive progress on emissions reductions and attract private sector investment. Investment in discovery research, as well as development and scaling-up of current prototypes, will increase the chances that new technologies not yet ready for commercial deployment, will be available in the future.
The UK has an opportunity to be a leader in certain low carbon technologies, services and systems that will be needed globally, with the government’s plan to Build Back Better focussing on the 3 pillars of infrastructure, skills and innovation. Innovation across key technology areas could contribute £60 billion in GVA through domestic and export activity annually in 2050[footnote 4]. Given the geographical distribution of the required activity, this in turn will help to level-up the country and mitigate the risks to jobs and the economy associated with moving away from current high-carbon sectors and help to build back better after the COVID-19 pandemic.
The global market for low carbon goods and services could be worth up to £1.8tn by 2030[footnote 5] and, within that, there is an opportunity for UK export sales of £60bn - £170bn[footnote 6], in areas such as electric vehicle manufacturing and supplying components for the wider electrification of transport, green finance, precision agriculture, renewables such as wind and heat pump technologies, sustainable construction and sustainable infrastructure including waste and water. There are other clear areas where, due to the UK’s current academic and business strengths, we could secure a leadership position in technologies if we act effectively including: advanced battery technologies, advanced photovoltaics, biomass and bioenergy, CCUS, floating offshore wind, fuel cell technologies, low carbon hydrogen/ammonia, machines and drives, power electronics and advanced nuclear reactor technologies.
International trade and investment are key to the promotion, growth and diffusion of UK green innovation at a global scale and for stimulating targeted inward investment to the UK from overseas. They also help to speed-up technological development, scale-up and dispersion, and drive down costs by enabling access to critical resources and increasing the returns to innovation by allowing access to larger markets. Research and innovation should aim to prioritise the scaling of commercial and exportable propositions for the UK alongside delivering carbon emissions reductions.
Prioritisation framework
Delivering net zero will require a wide-ranging portfolio of research and innovation support across the public sector, private sector and research communities. This Framework identifies the main challenges and key timelines over the next 5 to 10 years across each Carbon Budget and related sector as well as cross-cutting and systems-wide issues and linkages between sectors. It extends beyond technology to include research and innovation related to systems, processes, business models and the socio-economic considerations needed to encourage green choices. It will set the direction for publicly funded research and innovation towards net zero and aims to signal areas where new ideas, products and services have a potential market, to build confidence and catalyse research and investment from business, researchers and entrepreneurs. This research and innovation activity will start to narrow down options and ensure that we can invest at scale in the areas where this is needed for widespread deployment.
For government investment, we will prioritise spend based on:
- Expected contribution to delivering the UK’s carbon budgets and major decarbonisation – accelerating delivery of greenhouse gas emissions reductions by increasing certainty of technologies / solutions, including by taking into account the current state of technologies and the potential for research and innovation to make rapid progress;
- Building and maximising UK comparative advantage globally – focussing on areas with the highest potential for UK business and jobs. Developing and commercialising technologies, processes and business models for the energy transition can provide business opportunities and enhance economic competitiveness; and
- Retaining optionality of different net zero pathways – investing in a portfolio of solutions, and tolerating some failure, including novel technologies for areas such as greenhouse gas removals.
Both public research and innovation spending to push technology development and market-pull mechanisms such as policy, regulatory and financial frameworks will be needed for success.
We intend to subsequently publish a Delivery Plan which will show how these prioritisation principles have been applied and the current government programmes prioritised from this framework. Based on current understanding of technologies and solutions, the likely low regrets measures for investment over the next 5 to 10 years are:
Figure 3: Key low regrets areas for UK investment
Major decarbonisation opportunities
- Floating offshore wind
- Energy storage at scale and system flexibility - enablers of high renewables system
- Hydrogen - enabler of industrial fuel switching, heat and some negative emissions
- Carbon capture, utilisation and storage for industry - critical for hard to abate areas
- Buildings decarbonisation
- Land transport, including zero emission road vehicles, rail, light rail and active travel
- Aviation and maritime
- Agriculture and food
- Nature-based carbon removals, for example, afforestation, domestic perennial energy crops, short rotation forestry, and biochar.
Major business opportunities
- Transport - aviation, automotive, maritime
- Energy storage at scale
- Hydrogen
- Nuclear - Small Modular Reactors, Advanced Modular Reactors and advanced fuel cycle, particularly in export
- Offshore wind - with floating offshore wind potential new area for export and domestic deployment
Creates optionality in net zero pathways
- Energy efficiency
- Carbon capture, utilisation and storage - major enabler for industry, hydrogen and bioenegry with carbon capture and storage (BECCS)
- Innovation within industrial energy sectors - hard to abate and cannot be substituted by other technologies
- Sustainable land-use
- Negative emissions technologies including Direct Air Capture
The Framework will need to adapt and change over time given the current uncertainty on pathways to net zero and we will need to continue to invest in research for the longer-term solutions. We have not attempted to plan all the research and innovation needs over the next 30 years. Breakthrough technologies could revolutionise our understanding and approaches in some areas, although the time to develop and deploy new technologies across an economy means that the next decade – and the technologies we have available over that timescale – must not be wasted. Government, as well as business, will need to be agile and able to pivot plans in the future.
2. Funding for research and innovation
Publicly funded R&D
The International Energy Agency (IEA) ‘Net Zero by 2050’ report recommended that globally, government research and innovation spending needs to be increased and reprioritised. Technology areas, such as bioenergy, CCUS, electrification of buildings, industry and transport, and low carbon hydrogen, receive markedly less funding globally, in comparison to more established low carbon electricity generation and energy reduction and efficiency technologies.
The UK’s Innovation Strategy makes clear that the government continues to be committed to increasing direct public expenditure on research and innovation to a record £22bn per year and a key part of this will be net zero related. UK public funding for clean energy research and innovation has been on an upwards trajectory over the last decade as illustrated in Figure 4. Whilst this puts the UK in the top 10 OECD countries in terms of spend, it equates to just over 0.03% of GDP compared to countries such as France, Canada and Japan which are spending close to 0.05%[footnote 7] or above. Comparisons of spending levels and relative prioritisation of technologies by different countries can be seen in Figure 5.
Figure 4: UK government expenditure on energy innovation by technology group (£m)
Source: IEA R, D&D spending database.
Description of figure 4
Chart of UK government expenditure on energy innovation between 1985 and 2019 for 10 technology groups. Overall, expenditure decreases from 1985 to 2001, then steadily increases to 2019.
Figure 5: Total energy innovation and % spend by technology
Sources: IEA R,D&D Budget Database - data reflects 2019 R&D budgets, using 2020 prices; Mission Innovation Country Summaries
*China is not an IEA member - data is from Mission Innovation (2019)
**2018 IEA data is used
Description of figure 5
Bar chart representing the 2019 total energy innovation spend and its split across different technologies for 11 countries. Highest spend is the United Sates, then China; Japan; France; Germany; United Kingdom; Canada; Korea; Italy and Switzerland.
A greater proportion of funding is now needed for demonstrating and accelerating the commercialisation of technologies. The IEA estimates that technologies needed to deliver almost half of the CO2 reductions required to reach net zero by 2050 are still in prototype phases[footnote 8]. Given the urgency of delivering key new technologies and solutions to market, funding across early-stage discovery research, development of technologies and solutions and demonstration will need to increase. Within this the proportion focussed on demonstration is expected to significantly increase over the next 5 to 10 years as spend is rebalanced towards scaling-up technologies which will be needed to meet Carbon Budget 6.
Private sector R&D
Publicly funded R&D will not be sufficient on its own to deliver the step change in innovation needed. Private sector investment in research and innovation is essential and will help UK businesses to compete internationally and accelerate UK growth.
The UK has a vibrant ecosystem of innovative start-ups, with more ‘unicorns’ (start-ups that have grown to be valued $1 billion or more) than any other country in Europe[footnote 9] including a number operating in the energy sector. The Innovation Strategy sets out steps to foster this ecosystem including ensuring our research, development and innovation institutions serve the needs of businesses and places across the UK. This means ensuring firms can access the right private finance at the right stage, creating policy and regulatory frameworks which incentivise innovation, supporting businesses to commercialise new ideas, providing targeted public support where there are gaps in private markets and using government procurement as a lever to pull through innovation from idea to market.
Different green technologies and infrastructure will require different types of financial support depending on their maturity (see figures 6 and 7). We must engage all types of capital from early-stage grant and angel investment through to institutional finance like pension fund investors and inward investors from overseas.
Public funds will be used strategically to support new technologies, as well as emerging sectors, as they move from the research and innovation stage through to commercialisation and deployment. Early-stage research and innovation is supported by various government schemes, while later-stages can benefit from funds such as the Clean Growth Fund or support from the British Business Bank (BBB) in addition to other private investment sources.
For larger scale infrastructure finance, the government launched the UK Infrastructure Bank in June 2021. A combination of capital, government guarantees and private investment will enable more than £40bn of investment in areas most prone to market failure and to help deliver on its dual policy focus to tackle climate change and support regional and local economic growth.
Figure 6: Public Finance interventions across the different stages of commercialisation
Description of figure 6
Figure showing different types of financial support from start-up to commercialisation for new clean technologies and sectors.
Listed from more government support to less government support.
Start-ups / Corporate R&D > Research & development:
- Innovate UK
- UKRI
- NZIP
Start-Ups / R&D > Commercial prototype:
- Innovate UK
- NZIP
- Clean Growth Fund
- British Business Bank
- UKIB
Scale Up & Growth > Build & scale:
- Innovate UK
- British Business Bank
- UKIB
Scale Up & Growth > Proven commercial proposition:
- UKIB
- British Patient Capital
- UK Export Finance
Scale > Capital markets ready:
- British Patient Capital
- UK Export Finance
- London Stock Exchange
Supporting framework:
- Financing Green - Green Finance Institute, NS&I, Sovereign Green Board
- Green skills - UK Green Taxonomy
- Greening the financial system - UK Centre for Greening Finance & Investment, TCFD, TNFD
- NZ aligned regulation - FCA, Bank of England
Figure 7: Low carbon sectors commercial maturity and associated capital requirements
Description of figure 7
Figure mapping low carbon sectors onto graph from start-up to commercialisation and support available at each stage.
Listed from more government support to less government support. Sector financial independence increases from Start-ups to Scale. Financial risk / cost of capital decreases from Start-ups to Scale.
Start-ups / R&D > Research & development:
- Sector - Low Carbon Hydrogen
- Finance available - NZIP
- Funding organisations - Innovate UK
- Type of support - Grants
Start-Ups / R&D > Commercial prototype:
- Sector - Advanced Nuclear, CC&S
- Finance available - Clean Growth Fund, Revenue support: business models
- Funding organisations - Innovate UK
- Type of support - VC investment funds/ crowdfunding VCT/(S)EIS funds
Scale Up & Growth > Build & scale:
- Sector - CC&S, Long-term energy storage, Energy efficiency retrofits
- Finance available - Revenue support: business models
- Funding organisations - BBB, UKIB
- Type of support - Corporate ventures, Private equity, Infrastructure funds & Banks
Scale Up & Growth > Proven commercial proposition:
- Sector - Electrification of transport
- Finance available - Revenue support: business models
- Funding organisations - UKIB, Green Finance Institute
- Type of support - Corporate ventures, Private equity, Infrastructure funds & Banks
Scale > Capital markets ready:
- Sector - Offshore wind
- Finance available - Revenue support: business models
- Funding organisations - Green Finance Institute, London Stock Exchange
- Type of support - Institutional investors, for example, LGIM, HSBC, pension funds
Supporting framework:
- Financing Green - Sovereign Green Board, NS&I
- Green skills - UK Green Taxonomy
- Greening the financial system - UK Green Taxonomy, TCFD, TNFD
- NZ aligned regulation - FCA, Bank of England
International R&D Collaboration
International co-operation and collaboration, including access to research and innovation infrastructure, the sharing of experience and lessons learned and input to standards setting will also support the UK’s ability to meet its net zero target. Different countries have capabilities in different areas of the energy system and technology development and decisions on which areas of research and innovation to prioritise within the UK should consider whether the UK is best placed to ‘lead, collaborate or access’.
Mission Innovation is the primary international forum to strengthen cooperation on clean energy technology development, aiming to deliver a decade of innovation to make clean energy affordable and accessible for all. Combined, its members represent over 90% of global public sector investment in clean energy research and innovation. Now into its second phase, Mission Innovation 2.0 includes new international Missions, public-private innovation alliances aiming to accelerate tipping points in the cost and scale of clean energy solutions. The UK is co-lead for the Clean Hydrogen and Green Powered Future Missions and is a core member of the Zero Emission Shipping Mission. The UK also intends to associate to the Horizon Europe research and innovation funding programme which, along with participation in International Energy Agency Technology Collaboration Programmes, offers significant opportunities for knowledge sharing, network building and involvement in the development of future supply chain and market building.
The majority of emissions growth globally in the coming decades to 2050 is expected to be in developing and emerging economies as they raise standards of living. This is a key reason why the UK is also a major contributor to International Climate Finance, helping countries adopt low carbon pathways to meeting the energy needs of their populations and industries, as well as managing natural resources and adapting transport systems. Innovation plays a key role and the UK’s £1bn Ayrton Fund commitment aims to help drive forward the clean energy transition in developing countries by developing, testing and demonstrating innovative technologies and the business models to commercialise them. This will focus on the transformation of the whole energy system and work with developing countries on a series of priority challenges including industrial decarbonisation, sustainable cooling, efficient end-use appliances, modern cooking, smart energy, energy storage, next generation solar, and inclusive energy leaving no-one behind.
UK action on net zero innovation at home is critical if our 2050 target is to be met, but we also need to support action internationally if we are to pursue efforts to limit global temperature rises to 1.5°C. This Framework will support our on-going role in international initiatives and fora based on a clear outline of UK domestic net zero research and innovation interests, while our wider UK offer internationally also responds to the needs and opportunities of international markets and emerging economies.
3. A whole systems approach
An economy-wide transformation is required to reach net zero and a whole systems approach considers the interrelated nature of different sectors and between new technologies, consumer behaviour and business models. It considers the order in which different actions are required, and their cumulative impacts, and allows decision makers to assess interactions between different parts of the system and how these can combine to affect an outcome. New technologies, products and processes have the potential to influence other environmental outcomes, both positively and negatively. A systems approach can therefore help to identify potential co-benefits or tensions, manage uncertainty, target points of greatest leverage, mitigate unintended consequences, identify highest value and minimum cost pathways, maximise benefits and ensure an agile and dynamic approach to decision-making over time.
This Framework is a step towards implementing a whole systems approach to net zero research and innovation. Each chapter draws out the linkages between decarbonisation challenges and solutions in other sectors of the economy and identifies research and innovation needs that address system level issues. It considers both technological innovation and research into how people will respond to new technologies, services and business models. Research and innovation will also be needed to tackle key cross-cutting themes and system of systems questions. These will be important across many or all sectors and are developed in the table below.
These should be achieved whilst leaving the environment in a better state by improving biodiversity, air quality, water quality and availability, natural capital and resilience to climate change. Innovation to mitigate any wider environmental impacts of decarbonisation policies and proposals and harness any co-benefits from the transition will be important for wider government objectives. Improvements to measuring progress across the whole system will need to be developed. Looking beyond 2050, research into climate repair may become increasingly important, but is not considered further in this Framework which is focussed on activities that must happen now.
Research and innovation: whole-system challenges and needs
Navigating pathways to achieving net zero
Whole systems analysis is needed to understand net zero pathways and to address interdependencies and trade-offs across physical, natural, social and digital systems (including via deployment of technology, policies and processes).
- Cross-cutting research and a wide range of analytical tools, systems models and other systems-based approaches to explore the system dynamics and interactions between sectors in the transition to net zero.
- Assess the systemic effects of net zero interventions on GHG emissions and other environmental, social and economic outcomes. Develop tools and models to explore the broad, systemic impacts of alternative trajectories to net zero under different future scenarios including scenarios in which embedding resilience can mitigate the risk climate change poses to achieving net zero, for example where disruptions to natural systems may reduce GHG sequestration potential[footnote 10].
- Modelling to optimise the roles of different energy vectors.
- Systems research on the use of land for food production, biomass, and afforestation, among others, including analysis of interdependencies and trade-offs between multiple environmental outcomes and commitments, and economic and social goals.
- Feasibility and efficacy of large-scale GGR, including how this may inform decarbonisation pathways across sectors and where best to intervene.
- How decarbonisation interventions can deliver wider co-benefits in terms of environment and ecosystem services including air and water quality, noise, and health.
Managing socio-economic and behavioural impacts
Complex interactions between societal behaviours and new technology / policies need an understanding of system dynamics and how norms and green choices can both impact and be impacted by a net zero transition, and how more sustainable choices can be supported and incentivised.
- Attitudes towards low carbon technologies (such as hydrogen, CCUS, and GGRs) and measures to build consumer confidence and acceptance.
- Adoption of, and interaction with, smart technologies, including home energy use, electric vehicle charging and mobility services.
- Choices on purchasing and consuming more or less carbon-intensive goods, for example building materials and practices.
- How needs and preferences may change over time and in response to incentives, for example shifts to more active forms of travel.
- Innovation necessary for vulnerable end-users to participate in and benefit from the net zero transition.
- Role of advice in empowering end-users and tackling low levels of awareness and acceptance for low carbon interventions, for example energy reduction and efficiency.
Developing an enabling environment for net zero through new business models and finance
To support innovation across the economy, there is a need to develop new types of business models and financial mechanisms alongside clear standards to unlock the value of innovation, manage risk and enable faster uptake of low carbon solutions.
- New business models, standards and market arrangements to facilitate uptake of solutions, for example energy as a service and time-of-use tariffs.
- Green finance options to support new products and services.
- Economic models for new or significantly scaled-up commodities, for example hydrogen, CCUS and GGRs.
Taking a place-based approach
A successful net zero transition will be driven by locally and regionally appropriate solutions that vary across the UK. Cities, towns and neighbourhoods will be the locations where integrated cross-sector net zero solutions are delivered. Research and innovation is needed to support these objectives.
- Living-labs and local testbeds, including public / local engagement and participation in decision making into solutions, taking into account skills, employment, impacts, trade-offs and co-benefits to provide equitable and sustainable solutions.
- Design and retrofit buildings and the built environment, including towns and cities, to support more sustainable choices, including low carbon forms of travel.
- Optimise solutions at the local level, for example hydrogen heating where there is a local supply of low carbon hydrogen, distributed electricity generation / smart local energy systems, opportunities for making climate resilient homes / infrastructure as part net zero retrofits.
Mobilising digital solutions and data and the shift to Industry 4.0
Digital solutions and technologies, alongside adequate cyber and physical security, can support cross-sector integration, enable systems-level understanding and joined-up action and unlock resource and energy efficiency.
- Digital technologies to reduce energy and resource demand across sectors, for example reducing journey length or the need to travel.
- Availability and interoperability of data, including through standards and possibly blockchain based platforms, to enable active data flows throughout the energy and transport systems.
- Novel digital technologies, such as digital twins, to identify potential issues and inefficiencies and improve the cost effectiveness, efficiency, and flexibility of industry, buildings, and transport.
- Identify how and where digital technologies may increase energy demand, and measures to reduce energy requirements.
Developing an integrated energy system
The transition to net zero is driving fundamental changes to energy supply, demand, transmission, distribution, storage and use; trends that will be compounded by interlinkages between energy vectors and across different sectors of the economy. Research and innovation are needed to manage and integrate net zero energy systems.
- Enabling, and preparing for, flexibility across the energy value chain:
- Changes and opportunities that arise from a larger capacity, more dynamic electricity system, involving variable renewables alongside large-scale and long-term storage.
- Demand side response to increase system efficiencies.
- Interoperable smart appliances and flexibility services offering effective energy management / balancing, at building / home, local area and national levels.
- Market platforms across vectors to efficiently coordinate flexible and decentralised supply and demand.
- Identify energy needs across sectors and optimise existing and new infrastructure (including re-purposing where practical) to deliver energy in the most efficient ways:
- Energy vectors to promote system-wide efficiency.
- Impact of large-scale electrification of transport and buildings on the power system.
- Integration of transport and industry into local and national decarbonised energy system planning.
- Interactions between transport modes with wider systems and infrastructure.
- Optimising cogeneration potential and re-use of waste energy resources, for example using Combined Heat and Power (CHP) technology to minimise waste heat from power generation and other industrial processes.
- Research into appropriate economic, finance and business models to support delivery and identification of co-benefits.
- Demonstrating integrated energy-systems as well as technology-specific demonstrations.
Figure 8: An integrated approach to net zero: Simplified illustration of key system and sector interlinkages for the net zero transition
Description of figure 8
Figure representing an integrated approach to net zero. Five enablers (Data & interoperability; Finance; Policy; Skills; End-users) are seen to influence the 7 research and innovation challenges - Industry; CCUS & GGRs; Natural resources, waste and F-gases; Power; Transport; Heat and buildings; Hydrogen.
The following chapters include graphics centred on each sector, which further develop key sector interlinkages.
4. Research and innovation challenges
4.1 Power
Context
The power sector has led the UK’s efforts to reduce greenhouse gas emissions. In 1990, electricity generation accounted for 25% of UK GHG emissions, by 2019 this share had reduced to 13%[footnote 11]. In 1990, fossil fuels provided nearly 80% of electricity supply. Today, over half the UK’s electricity comes from low carbon sources and the average carbon intensity has fallen by more than 50% from 1998 levels[footnote 12].
This rapid decarbonisation will need to accelerate if the UK is to reach net zero by 2050. The Energy White Paper sets out the government’s policies and commitments for achieving net zero in the energy sector with the ambition now updated to have decarbonised the UK electricity system by 2035. This includes 40GW of offshore wind by 2030 (a quadrupling of current capacity that is estimated to support 60,000 jobs), supporting the development of the next generation of nuclear technology, building world-leading digital infrastructure for the energy system, and a commitment to assess market changes that may be required to facilitate and encourage the development and uptake of innovative tariffs and products.[footnote 13]
Substantial electrification of surface transport and heat for buildings means that electricity demand is likely to at least double by 2050[footnote 14] (see Figure 9). In future, it is likely that the majority of UK electricity generation will come from wind and solar, with the remainder from a mix of nuclear, bioenergy with carbon capture & storage (BECCS), gas with CCS (see sections 4.3), hydrogen (see sections 4.2) and other renewables such as hydro and tidal power.
Figure 9: Electricity mix today and illustrative 2050 electricity mixes
Source: Energy Trends, table 5.1 and 6.1; BEIS analysis
Description of figure 9
Bar chart representing the UK’s electricity mix in 2019 and a prediction of the mix for Great Britain in 2050. The electricity demand could double as other sectors electrify and be met by a range of generation mixes.
As variable and distributed technologies take up an increasing share of supply, the wider electricity system must undergo a parallel transformation with supporting policy changes and infrastructure improvements. Energy sector and other companies will need to invest in transmission and distribution system innovation (including interconnection), long-duration storage, demand reduction and demand-side response - all whilst keeping costs to end-users as low as possible and maximising energy efficiency.
Research and innovation in the power sector also presents significant opportunities for international collaboration and UK leadership. The UK is co-leading the Green Powered Future Mission[footnote 15] which aims to demonstrate cost-efficient integration of up to 100% variable renewable energy and is leading efforts to create standardised data systems and digital platforms that enable interoperability and cross-sectoral flexibility to deliver fully integrated power systems across the world.
Business opportunities for UK companies exist in a number of areas, including developing business models for flexible markets, billing services and engagement models. The UK also has one of the largest markets for offshore wind and is at the leading edge of global development in areas such as new nuclear, smart technologies and tidal. Opportunities exist to continue to develop expertise and reduce costs across these sectors, to supply both domestic and export markets and integrate UK companies into international supply chains.
Figure 10: System interlinkages between Power and other sectors
Description of figure 10
Figure representing the system interlinkages between Power and the 6 other sectors:
Natural resources, Waste and F-gases -
- Biomass supply for bioenergy, including BECCS
- Electrification of agricultural equipment
Transport -
- Power demand for all types of electric vehicles
- Vehicle-to-X technologies
- Low-carbon transport of goods
Heat and buildings -
- Electrification of heat (heat pumps and heat networks)
- Smart home technologies & appliances
- Demand side response for grid balancing
Hydrogen -
- Hydrogen production via electrolysis
- Power generation from hydrogen (such as peaking)
- Hydrogen appliances and vehicles
Industry -
- Electrification of industrial processes
- Heat networks
- Manufacture of low- carbon equipment and components
CCUS & GGRs -
- Power generation via BECCS and gas with CCUS
- Power demand from DACs
4.1.1 System integration and flexibility
Research and Innovation: challenges and needs
A flexible energy system is essential for integrating high volumes kf low carbon power, heat and transport. The Smart Systems and Flexibility Plan 2021 sets out how government and industry will transition to a smart, flexible, decarbonised energy system[footnote 16]. Technologies such as energy storage, smart charging of electric vehicles, flexible heating systems and interconnection could save up to £10 billion per year by 2050[footnote 17] by reducing the amount of generation and network infrastructure needed to decarbonise. To date, power system flexibility has largely been provided by fossil fuels, as we turn up or turn down coal or gas fired power stations. In the future, we need an energy system that matches new sources of demand to renewable generation by using low carbon flexibility across the system underpinned by smart, secure, data-enabled technologies to ensure reliability and interoperability.
Research and innovation can help inform policy and regulation in this context, for example through market arrangements to align price signals with the actual cost of generation at a given point in time, which will help to unlock the potential of flexible technologies. New business models and smart technologies, informed by behavioural research, will give end-users the opportunity to match consumption patterns to times of cheap low carbon electricity and gain greater control over their energy usage. Customer-focussed innovation across the supply chain, including industrial energy users as well as domestic consumers, will be key to this transformation.
Demonstrating flexibility at scale in the domestic market and effectively leveraging strengths in information technology, artificial intelligence, financial settlement, aggregation, and advisory services can help to strengthen UK leadership in system integration and flexibility.
Accelerating the transition to an interoperable, digitalised, cyber-secure system
Develop solutions to increase grid operators’ visibility and awareness of energy assets (such as electric vehicles (EVs), EV charge points and heat pumps) - leading to faster, more complete, more accurate registration data available to energy networks.
Increase in availability and interoperability of data, including through standards to enable active data flows throughout the system, building on existing infrastructure including smart metering capability, while providing adequate data privacy and security.
Improve interoperability and cyber security of smart appliances and flexibility services for end-users, including through standards to enable technology types to interact and operate with service providers.
Understanding, enabling and demonstrating flexible demand
Develop information and communication technology platforms capable of coordinating Demand Side Response across distributed assets, including through standards for interoperability.
Demonstrate the flexible operation of various smart technologies in buildings of all scales.
Develop intelligent and/or autonomous devices and approaches for end-users to deliver flexibility.
Facilitate and encourage consumer uptake of flexibility services, including:
- Developing compelling and effective solutions (including products, services, and business models) for a diverse range of end-users including vulnerable end-users.
- Developing and enabling energy suppliers to develop products and services which use intelligence and automation to improve and simplify the consumer experience of engaging with flexibility services.
- Improve understanding of consumer behaviour and willingness to shift electricity use (including EV charging, vehicle to grid and smart appliance use) in response to dynamic time-of-use tariffs, price signals and other incentives through the use of smart technologies, such as smart meters.
- Investigate the impact of exported energy-based tariff adjustments, incentivising behaviour that facilitates system optimisation.
Enabling, developing and demonstrating energy storage particularly at large scales
Improve energy storage performance and cost, including:
- Stationary battery technology where improvements will not be driven by EV uptake, with particular focus on battery management and manufacture.
- Immature bulk storage options (such as sodium ion battery technologies).
- Develop Vehicle-to-X energy technologies.
- Research, development and demonstration of novel energy storage solutions for a range of durations and at appropriate storage and power capacity. This could include large-scale hydrogen storage, hydrogen fuel cells, gravitational solutions, compressed air storage, flywheels and other mechanical storage systems, supercapacitors, thermal and chemical options (including molten salts, green ammonia, etc.).
- Develop strategies for integrating various types of storage into the grid, including understanding whether storage is best at the edge or centre of networks.
Developing and demonstrating flexible platforms for smarter markets
Develop market platforms to efficiently coordinate flexible and decentralised supply and demand, that is enabling multiple buyers (suppliers, networks, system operators) and multiple sellers (end-users, aggregators, local communities) to participate across markets, driving an optimised energy system, innovation, competition and maximising consumer participation.
Demonstrate platforms at multiple levels of aggregation including improved interaction between transmission and distribution system operators and linking to other energy vectors as well as secondary trading of flexibility.
Prepare networks for the energy system transformation and integration
Understand and develop collaborative solutions to manage the changes that arise from a larger capacity, more dynamic system with high penetration of variable renewable energy, including:
- Solutions that manage network or system peaks and allow better utilisation from a variety of assets such as grid-connected low carbon technologies and in-home flexible use of heating and EV smart charging.
- Lower-cost innovative options for increasing the capacity of the low voltage distribution network to meet increased electricity demand, this includes low voltage smart load management and control systems as well as demand side options.
- Whole systems integration for future energy provisions around heat, power and transport and improve coordination between networks and other system participants and ancillary services across local, national and international scales.
4.1.2 Renewables
Research and Innovation: challenges and needs
Renewable generation will need to be rapidly deployed to reach net zero. The offshore wind industry has developed quickly in the last decade. Large-scale deployment, supported through the government’s Contracts for Difference (CfD) scheme, has driven innovation across the supply chain. For this trajectory to continue and expand globally, innovation must enable turbines to be deployed in deeper waters and floating offshore wind is likely to be key to unlocking these locations. Innovations in fixed bottom offshore wind will also be important including in wind turbine foundation design, as well those that decrease the costs of foundation manufacture and installations. Other renewables, such as solar photovoltaics (PV) at domestic and community scales, are expected to make contributions to our energy mix. UK companies could be at the forefront of technological advancements that will drive up panel efficiencies and integrate with other products and services. This research and innovation will be delivered predominantly through private sector investment, although support for next generation solar photovoltaics at earlier technology readiness levels (TRLs) will be needed. Research and innovation can also help unlock new opportunities in other early stage TRL technologies, such as tidal and wave generation.
Accelerating the deployment of offshore wind capacity
Contribute to global development of next generation turbines by focussing on key areas of potential UK competitive advantage including gearboxes, drive trains, generators, new materials and foundation design.
Further innovation to:
- Improve grid integration to smooth the variability of wind energy and decrease the costs of transmission, including long distance AC transmission, high voltage DC transmission, novel methods of power transmission, and advanced wind modelling.
- Reduce costs via larger turbines, robotics and artificial intelligence.
- Improve cable burial and repair, logistics, installation, and smart and remote operations and maintenance.
- Improve power conversion technologies for offshore grid networks.
Unlocking deep water offshore wind sites deeper than 50 meters
Research and innovation to improve floating offshore wind technology including:
- Foundations
- Dynamic cable design / modelling
- Novel installation and mooring systems and logistics
- Offshore operations
Further improve fixed bottom offshore wind to decrease the costs of foundation manufacture and installation.
Mitigating the wider impacts from wind turbine installation and operation (both fixed and floating)
Maintain the effective surveillance of airspace by developing technologies that will allow co-existence of radar and offshore wind farms.
Develop technological solutions for mitigation and compensation for cumulative environmental effects, including underwater noise effects on marine mammals, the effects of cabling on benthic habitats, the effects of bird collision and displacement and end of life recycling of structures.
Developing and demonstrating earlier-stage renewables including next generation PV, tidal and other lower TRL technologies
Develop and demonstrate that solar technologies can operate reliably, at scale, and at competitive cost through research and innovation in the following areas:
- Next-generation solar PV including new or modified cell chemistries; inverters with increased efficiency and lifetimes.
- Building Integrated PV (BIPV) working with construction sector. Research and innovation for more speculative solar technologies and conversion processes.
Demonstrate the potential for tidal and wave technologies to operate reliably, at scale, and at competitive cost through research and innovation in the following areas:
- Turbine blades, including material innovation (such as concrete and polymers) and novel structure design.
- Power take-off and control technologies, foundations, moorings, operations and maintenance.
- Understanding wave and tidal energy resource through resource modelling innovation.
Research and innovation for more speculative renewable technologies and conversion processes.
4.1.3 Nuclear
Research and Innovation: challenges and needs
Nuclear fission technologies can deliver reliable low carbon electricity to compensate for the variable output of renewables. Most technology development is centred around 2 broad technology categories - Small Modular Reactors (SMRs) and Advanced Modular Reactors (AMRs). Innovation is also required in the way that nuclear technologies convert their energy into usable applications of reliable low carbon electricity, low carbon hydrogen, and heat.
SMRs are usually based on proven water-cooled reactors similar to current nuclear power station reactors, but on a smaller scale. Components can be manufactured in factories using innovative techniques and then transported to site to be assembled. AMRs are next generation reactors which use novel cooling systems or fuels and may offer new functionalities (such as high temperature heat that could be used to decarbonise industrial processes). These reactors could operate at over 800°C and unlock efficient production of low carbon hydrogen and synthetic fuels.
There is a related policy need to assure investors and developers that nuclear technologies and developments are investable and that credible routes to market will exist.
Develop Small Modular Reactors (SMRs)
Develop first of a kind SMR by the 2030s.
Deliver and demonstrate innovative engineering design, materials, manufacturing and deployment methods to reduce costs and risk to SMRs supporting decarbonisation in all technical and economically viable ways.
Develop and demonstrate Advanced Modular Reactors (AMRs)
Demonstrate AMR technology in the UK by early 2030s.
Identify optimum operating temperature to maximise the value of AMRs in a decarbonised energy system.
Deliver AMR design and supporting research and development needs including materials, modelling and simulation validation, modular and factory build, and advanced joining and construction.
Develop and deliver AMR fuel by developing the design, demonstrating its performance and proving the fuel cycle.
Integrate advanced nuclear with other technologies to support a flexible energy system
Demonstrate the system integration of nuclear to support a flexible energy system, including understanding and demonstrating:
- electricity supply either through directly varying electrical output (potentially alongside cogeneration by diverting energy output to other activities) or via energy storage in the form of hydrogen and heat storage systems
- process heat to decarbonise industry
- feasibility of heat use for direct air capture of carbon dioxide
- nuclear potential for district heating
Driving continual improvement in large-scale nuclear
Demonstrate the role of Accident Tolerant Fuel to enhance safety margins of existing technology.
Innovation in project delivery models to reduce risk and cost of new deployment.
Demonstrate ability of large scale nuclear to support flexible electricity, hydrogen and heat provision, complementing other energy technologies and supporting wider integrated energy system decarbonisation.
Demonstrate the cost reduction potential through repeatability of design and application of digital and advanced manufacturing and modular approaches.
Research and development in nuclear fusion with a view to options beyond 2050
Design and build a prototype fusion power demonstrator in the UK by the 2040s to demonstrate the commercial viability of fusion.
Improving processes for decommissioning and waste
Research and development aligned to possible future fuel cycle scenarios.
4.1.4 Bioenergy and BECCS
Research and Innovation: challenges and needs
Sustainable biomass has a range of applications as a substitute for fossil-fuel based products and activities. Coupling bioenergy with CCS to deliver negative emissions (BECCS) also makes biomass an option for reaching net zero emissions. Biomass already plays a prominent role in the UK energy mix. The conversion of biomass and other organic wastes and residues into fuels met around 10.5% of primary energy demand in 2020[footnote 18]. The versatility of biomass means that it has the potential to provide decarbonisation options in most sectors of the economy including hydrogen production, industry, renewable transport fuels for aviation and surface transport (addressed in respective chapters) and power generation with CCS.
The UK will need significant amounts of bioenergy to achieve net zero[footnote 19]. The sustainable sourcing of biomass feedstocks from forestry, agriculture and waste is critical and raises economic, environmental, social, and land-use challenges, all of which require research if the UK is to increase the use of imported or domestic and home-grown biomass (see also section 4.6.3).
Whole systems approach to bioenergy
Identify the most cost effective and GHG-optimal methods of utilising sustainable biomass to meet net zero, taking into account technical limitations for different biomass feedstocks, where the energy vectors will be needed, where the biomass materials will be sourced, and compare with counterfactual production routes.
Creating and securing a sustainable and reliable supply of quality biomass
(Note that biomass production is covered in section 4.6.3).
Address environmental and other impacts (such as GHG, air quality, biodiversity and water use) of aggregating and scaling up sustainable biomass supply chains, for both imported and domestic biomass.
Improvements in biomass productivity, through breeding, planting, cultivating, harvesting and pre-processing.
Improving the performance and commercial viability of gasification conversion technologies
Research into flexible gasification systems that can handle various feedstocks and understand the potential domestic biomass resource that is suitable for gasification.
Research into flexible gasification systems that can efficiently produce various end products (for example, sustainable aviation fuel, hydrogen, biomethane).
Improve syngas treatment / clean-up technologies to improve gasification plant availability at various scales.
Support innovation to enable a fully integrated, scaled-up advanced gasification demonstration plant.
Enable full integration of advanced gasification flowsheet through a first of a kind plant. This is to demonstrate performance and provide investor assurance for commercialisation.
Exploring routes to deploy BECCS
(See also sections 4.2.1, 4.3 and 4.6.3).
Research into suitable sustainable feedstocks and pre-processing technologies that deliver the greatest decarbonisation and negative emission potential in different end uses (for example, power, transport, industry).
Research to assess the environmental and public health consequences of these approaches, such as for air quality, and mitigation options to address these.
Figure 11: Power research and innovation needs timeline
System integration and flexibility
Accelerating the transition to an interoperable, digitalised, cyber-secure system:
- Develop solutions to increase grid operators’ visibility and awareness of energy assets (such as EVs, EV chargepoints and heat pumps) - short term (2020 to 2025)
- Increase availability and interoperability of data, including through standards to enable active data flows throughout the system - short to medium term (2020 to 2030)
- Improve interoperability and cyber security of smart appliances and flexibility services for end-users, including through standards- short term (2020 to 2025)
Understanding, enabling and demonstrating flexible demand:
- Develop information and communication technology platforms capable of coordinating DSR across distributed assets - short to medium term (2020 to 2030)
- Demonstrate the flexible operation of various smart technologies in buildings of all scales - short to medium term (2020 to 2030)
- Develop sophisticated, intelligent and / or autonomous devices and approaches for end-users to deliver flexibility - short to medium term (2020 to 2030)
- Develop compelling and effective solutions (products, services, business models) for a diverse range of end-users, including low income / vulnerable end-users - short to longer term (2020 to 2030s and beyond)
- Develop and enable energy suppliers to develop products and services which use intelligence and automation to improve and simplify the consumer experience - short to longer term (2020 to 2030s and beyond)
- Improve understanding of consumer behaviour and willingness to shift electricity use in response to time-of-use tariffs, price signals or other incentives through the use of smart technologies - short to longer term (2020 to 2030s and beyond)
- Investigate the impact of exported energy-based tariff adjustments, incentivising behaviour that facilitates system optimisation - short to longer term (2020 to 2030s and beyond)
Enabling, developing and demonstrating energy storage, particularly at large-scale:
- Improve energy storage performance and cost, including for stationary battery technology, with particular focus on battery management and manufacture - short to medium term (2020 to 2030)
- Improve energy storage performance and cost for immature bulk storage options (such as sodium ion battery technologies) - short to medium term (2020 to 2030)
- Develop vehicle-to-X energy technologies - short to medium term (2020 to 2030)
- Research, development and demonstration of novel energy to electricity storage solutions for a range of durations and at appropriate storage and power capacity (such as electrolytic hydrogen, hydrogen fuel cells, gravitational solutions etc) - short to medium term (2020 to 2030)
- Develop strategies for integrating various types of storage in the grid, including whether storage is best at the edge or centre of the network - short to medium term (2020 to 2030)
Developing and demonstrating flexible platforms for smarter markets:
- Develop market platforms to efficiently coordinate flexible and decentralised supply and demand - short term (2020 to 2025)
- Demonstrate platforms at multiple levels of aggregation (including improved interaction between transmission and distribution system operators) and linking to other energy vectors as well as secondary trading of flexibility - short to medium term (2020 to 2030)
Prepare networks for the energy system transformation and integration:
- Understand and develop solutions to manage changes from a larger capacity, more dynamic system with high penetration of variable renewable energy - short to medium term (2020 to 2030)
- Innovations for solutions that manage network or system peaks and allow better utilisation from a variety of assets - short to medium term (2020 to 2030)
- Lower-cost innovative options for increasing the capacity of the low voltage distribution network to meet increased electricity demands - short to medium term (2020 to 2030)
- Research into whole systems integration for future energy provisions around heat, power and transport and improve coordination between networks and other system participants across local, national and international systems - short to longer term (2020 to 2030s and beyond)
Policy Ambitions:
- 40GW of offshore wind, including 1GW floating offshore wind - by 2030
- Advanced Modular Reactors demonstrator - by the early 2030s
- Prototype fusion power demonstrator - by the 2040s
- Deploy at least one operational power CCUS project - by 2030
- Deploy first of a kind SMR - by the 2030s
- Publish Biomass Strategy to establish the role of BECCS - by 2022
Renewables
Accelerating the deployment of offshore wind capacity:
- Develop next generation turbines, focussing on areas of UK advantage including gearboxes, drive trains, generators, new materials and foundation design - short to longer term (2020 to 2030s and beyond)
- Innovation to improve grid integration to smooth the variability of wind energy and decrease the costs of transmission (including long distance AC transmission, high voltage DC transmission) - short term (2020 to 2025)
- Innovation to reduce costs via larger turbines, robotics and Artificial Intelligence - medium term (2025 to 2030)
- Innovation to improve cable burial and repair, logistics, installation, and smart and remote operations and maintenance - short to medium term (2020 to 2030)
- Innovation to improve power conversion technologies for offshore grid networks - medium term (2025 to 2030)
Unlocking deep water offshore wind sites deeper than 50 meters:
- Research and innovation to improve floating offshore wind technology including:
- Foundations - short term (2020 to 2025)
- Dynamic cable design/modelling - short term (2020 to 2025)
- Novel installation and mooring systems and logistics - short term (2020 to 2025)
- Offshore operations - short term (2020 to 2025)
- Further improve fixed bottom offshore wind to decrease the costs of foundation manufacture and installation - short term (2020 to 2025)
Mitigating wider impacts from wind turbines (fixed and floating):
- Maintain effective surveillance of airspace by developing technologies that will allow co-existence of radar and offshore wind farms - short to longer term (2020 to 2030s and beyond)
- Development of technological solutions for mitigation and compensation for cumulative environmental effects - short to longer term (2020 to 2030s and beyond)
Developing and demonstrating earlier-stage renewables including next generation PV, tidal and lower TRL technologies:
- Solar Technologies: research and innovation in next-generation solar PV including new or modified cell chemistries; inverters with increases in efficiency and lifetimes - short to medium term (2020 to 2030)
- Solar Technologies: research and innovation in Building Integrated PV (BIPV) working with construction sector - short to medium term (2020 to 2030)
- Research and innovation for more speculative solar technologies and conversion processes - short to medium term (2020 to 2030)
- Tidal technologies: innovation to improve turbine blades, material innovation and novel structure design - medium term (2025 to 2030)
- Tidal technologies: improve power take-off and control technologies, foundations, moorings, operations and maintenance - medium term (2025 to 2030)
- Improve understanding of wave and tidal energy resource through resource modelling innovation - medium term (2025 to 2030)
- Other and lower TRL technologies: Research and innovation for more speculative renewable technologies and conversion processes - short to medium term (2020 to 2030)
Nuclear
Develop Small Modular Reactors (SMRs):
- Develop first-of-a-kind SMR by the 2030s - short to medium term (2020 to 2030)
- Deliver and demonstrate innovative engineering design, materials, manufacturing and deployment methods to reduce costs and risk to SMRs supporting decarbonisation - short term (2020 to 2025)
Develop and demonstrate Advanced Modular Reactors (AMRs):
- Demonstrate AMR technology in the UK by early 2030’s - short to medium term (2020 to 2030)
- Identify optimum operating temperature to maximise the value of AMRs in a decarbonised energy system - short to medium term (2020 to 2030)
- Deliver AMR design and supporting research and development needs, including materials, modelling and simulation validation, modular and factory build, advanced joining and construction - short to medium term (2020 to 2027)
- Develop and deliver AMR fuel by developing the design, demonstrating its performance and proving the fuel cycle - short term (2020 to 2025)
Integrate advanced nuclear with other technologies to support a flexible energy system:
- Demonstrate the system integration of nuclear to support a flexible energy system, including understanding and demonstrating: electricity supply either through directly varying electrical output (potentially alongside cogeneration) or via energy storage in the form of hydrogen and heat storage systems - medium term (2025 to 2030)
- Develop and demonstrate the technologies for nuclear process heat to decarbonise industry including sustainable fuel manufacture, ammonia production, direct air capture systems and traditional manufacture of carbon intensive industries such as paper, steel and glass - medium term (2025 to 2030)
- Demonstrate and underpin the role for nuclear heat to energise zero carbon district heating systems - medium term (2025 to 2030)
Driving continual improvement in large-scale nuclear:
- Demonstrate the role of Accident Tolerant Fuel to enhance safety margins of existing technology - medium term (2025 to 2030)
- Innovation in project delivery models to reduce risk and cost of new deployment - medium term (2025 to 2030)
- Demonstrate ability of large scale nuclear to support flexible electricity, hydrogen and heat provision - short to medium term (2020 to 2030)
- Demonstrate the cost reduction potential through repeatability of design and application of digital and advanced manufacturing and modular approaches - short to medium term (2020 to 2030)
Research and development in nuclear fusion with a view to options beyond 2050:
- Design and build a prototype fusion power demonstrator in the UK by the 2040s to demonstrate the commercial viability of fusion - short to longer term (2020 to 2030s and beyond)
Improving processes for decommissioning and waste:
- Research and development aligned to possible future fuel cycle scenarios - short to medium term (2020 to 2030)
Bioenergy and BECCS
Whole systems approach to bioenergy in meeting net zero:
- Identify the most cost effective and GHG-optimal methods of utilising sustainable biomass to meet net zero - short term (2020 to 2025)
Creating and securing a sustainable and reliable supply of quality biomass:
- Address environmental (greenhouse gas, air quality, biodiversity, water use) and other challenges of aggregating and scaling up sustainable biomass supply chains, for both imported and domestic biomass - medium term (2025 to 2030)
- Improvements in biomass production and pre-processing, including woody biomass, agricultural residues, waste, perennial energy crops and marine feedstocks to make them suitable for a greater variety of end uses, reduce supply chain losses and maximise their GHG emission reduction potential - short term (2020 to 2025)
Improving the performance and commercial viability of gasification conversion technologies:
- Research into flexible gasification systems that can handle various feedstocks, and understand the potential domestic biomass resource that is suitable for gasification - short term (2020 to 2025)
- Research into flexible gasification systems that can efficiently produce various end products (for example, sustainable aviation fuel, hydrogen, biomethane) - short to medium term (2020 to 2030)
- Improve syngas treatment / clean-up technologies to improve gasification plant availability at various scales - short to medium term (2020 to 2030)
- Support innovation to enable a fully integrated, scale-up advanced gasification demonstration plant - short to medium term (2020 to 2030)
- Enable full integration of advanced gasification flowsheet through a first of a kind plant - short to medium term (2020 to 2030)
Exploring routes to deploy BECCS:
- Research into suitable sustainable feedstocks and pre-processing technologies that deliver greatest decarbonisation and negative emission potential in different end uses (for example, power, transport, and industry) - short term (2020 to 2025)
- Research to assess the environmental and public health consequences of these approaches, such as for air quality, and mitigation options to address these - short term (2020 to 2025)
4.2 Industry and low carbon hydrogen supply
4.2.1 Transitioning to a net zero industrial base
Context
Industry contributes £180bn per year to the UK economy, accounting for 8% of UK GDP and providing 2.5m direct jobs[footnote 20] as well as 5m jobs across the value chain[footnote 21]. It is, however, also a major source of CO2 and other GHGs, and high emitting sectors include iron and steel, chemicals, petrochemicals (plastics) and cement and lime. In 2019, direct emissions from manufacturing and refineries accounted for around 16% (71 MtCO2e) of total UK GHG emissions[footnote 22]. Over half of these come from the UK’s 6 major heavy industry ‘clusters’ – Grangemouth, Humberside, Merseyside, Southampton, South Wales and Teesside. The bulk of industry emissions result from direct fossil fuel combustion for heat, but process emissions from chemical reactions (such as to produce cement or iron) also contribute.
Since 1990, total UK Industry emissions have more than halved mainly due to the changing structure of the UK’s manufacturing sector, improved energy efficiency and a shift to lower carbon fuels. However, despite this progress, the overall pace of reduction is slowing and critical technologies required for further emissions reductions, such as electrification, low carbon hydrogen, bioenergy and Carbon Capture, Utilisation and Storage (CCUS), remain globally underfunded in comparison to more established low carbon electricity generation and energy reduction and efficiency technologies[footnote 23]. Heavy industry is one of the hardest-to-abate sectors and many technologies to reduce emissions are still at an early stage of development.
By 2050, emissions from industry need to fall by at least 90% with all remaining emissions offset by GHG removals (see section 4.3). The Industrial Decarbonisation Strategy[footnote 24] and the Net Zero Strategy set out a framework for how government and industry can work together to achieve decarbonisation targets whilst also capitalising on clean growth opportunities. They highlight the following key milestones and targets:
- Connecting 2 of the UK’s major industrial clusters to CCS decarbonisation infrastructure by the mid-2020s and 4 by 2030.
- Fuel switching to low carbon hydrogen, which has the potential to increase the abatement of industrial emissions from 9 MtCO2e to 11 MtCO2e by 2035.
- Establishing the world’s first net zero industrial cluster and related systems by 2040.
- Almost no fossil fuel in use by 2050 unless combined with carbon capture.
The next decade will be critical for laying the policy and infrastructure foundations for deep decarbonisation whilst ensuring a range of decarbonisation options are available to industry by 2030. The 2020s are also critical to reach maximum energy and resource efficiency levels before more expensive decarbonisation options are rolled out from the 2030s onwards. At that point, deep decarbonisation must become the norm across UK industry. A range of technologies are required to reduce emissions across different industry sectors and sites. Broadly, these fall into 3 categories:
- Resource and energy efficiency
- Switching to low carbon fuels, such as electricity, hydrogen and bioenergy
- Carbon Capture, Utilisation and Storage (CCUS) for industrial emissions
There will be no universal solution and a multi-faceted approach to innovation and wider deployment is required with a need to evaluate and experiment. Environmental and pollution control regulation will also need to adapt for new technologies so that decarbonisation of industry delivers co-benefits for other objectives such as air quality. Alongside technology development, there is a need to strengthen the market for low carbon industrial goods as well as improving transparency on embodied emissions. Mechanisms such as carbon pricing and getting end-users to choose low carbon products and services will be important, including through data transparency, product labelling, public procurement and partnerships with the private sector.
The UK is well positioned to expand its low carbon exports related to industrial decarbonisation. This can build on the UK’s existing strong competitive position in fields such as biochemicals (possibly in the future utilising CO2 from CCS), data analytics and process optimisation, but there are also likely to be new opportunities to develop knowledge and technologies covering the full range of UK industry sectors: metals and minerals, chemicals, food and drink, paper and pulp, ceramics, glass, oil refineries and less energy intensive manufacturing. Significant opportunities are also likely in emerging industries, including low carbon hydrogen and CCUS.
Figure 12: System interlinkages between Industry and other sectors
Description of figure 12
Figure representing the system interlinkages between Industry and the 6 other sectors:
Natural resources, Waste and F-gases -
- Bioenergy used to fuel industrial processes and BECCS
- Biomass supply for industries, for example, paper, food, construction
- Circular economy & material flows
Transport -
- Co-located hydrogen hubs
- Manufacture of low carbon vehicles and components
- Transport of goods and services
- Low carbon materials
Heat and buildings -
- Hydrogen for buildings heat near to industrial clusters
- Supply of low carbon building materials
Hydrogen -
- Hydrogen as fuel for industrial processes
- Hydrogen production and storage co-located with industrial clusters
Power -
- Electrification of industrial processes
- Heat networks
- Manufacture of low-carbon equipment and components
CCUS & GGRs -
- CCUS applied to industrial processes
- CO2 transport and storage infrastructure focussed on industrial clusters
Research and innovation: challenges and needs
Research and innovation is needed this decade to identify and trial solutions which are best suited to different industries and contexts and to reach maximum energy and resource efficiency levels before more expensive decarbonisation options are rolled out from the 2030s onwards. From then, deep decarbonisation must become the norm across UK industry.
Improving resource and energy efficiency
In addition to policies that encourage accelerated uptake of existing technologies, innovation in resource and energy efficiency is needed in all parts of the manufacturing supply chain.
Reduce the impact of extraction and use of raw materials. Development of alternative, renewable feedstocks, along with a step change in the use of recycled material into new products is needed. For example:
- Research on potential for the chemical sector to use biological feedstocks to replace existing products and produce new (for example, bio-degradable) products and materials.
- Low carbon material inputs such as lower clinker cements and concretes, alternative binders and cement formulations.
More resource efficient manufacturing processes. New technologies and methods for manufacture of existing and new materials are needed, these include:
- Advanced technologies and new manufacturing processes that can provide a step-change in emissions reductions, such as new steelmaking technologies including electrolysis of iron ore to produce ‘green steel’.
- Research and testing related to new heat recovery techniques.
- Advanced manufacturing technologies, such as near net shaped and additive manufacturing (or 3D printing) to create lighter, cheaper and less resource intensive materials, reduce lead times and extend product life cycles.
- Digital Twin technology to identify potential issues and inefficiencies and improve the cost effectiveness, efficiency and flexibility of production.
Reduce the impact of a product in use. Redesigning a product to have a lower environmental impact including:
- Light weighting to reduce the weight of material inputs into a product.
- Using eco-design to design products for disassembly, remanufacture or recycling of end-of-life parts or products so that they can return to like-new or better performance, or new products that can be made with reused / recycled and alternative materials.
- Increasing product lifetime or switching to / creating re-useable products where possible.
End of life. Minimise the loss of materials at the end of life and prevent the impact of any waste on the environment. For example:
- Business model innovation (leasing, product service systems or pay as you go) that supports more efficient use of resources.
- Advanced separation and sorting of waste that enables more and higher quality recycling.
- Design and produce biodegradable and compostable materials with properties comparable to difficult to recycle materials, such as engineering plastics.
Switching to low and zero-carbon fuels (such as electricity, hydrogen and biomass)
Innovation is needed in a wide range of lower carbon fuels (including hydrogen, electricity and bioenergy), as well as ancillary technologies to enable fuel switching, such as metering of new fuels and site infrastructure. Support needs to take into account wider environmental goals, such as improving air and water quality, and necessary innovation in abatement technologies for pollutant emissions from new fuels.
For hydrogen:
- Demonstrate low carbon hydrogen as a feedstock for industrial sectors, new products and synfuels.
- Technologies for equipment categories where there is a potential for significant demand for hydrogen, including industrial boilers and combined heat and power systems (CHPs).
- Technologies for high temperature direct firing, for example, primary steel production, glass, ceramics and chemicals.
- Innovation to connect dispersed sites to hydrogen networks in industrial clusters, for example, repurposing parts of existing natural gas grid.
- Innovation to make existing gas equipment ‘hydrogen-ready’.
- Innovation to reduce costs to support low carbon hydrogen being competitively priced.
For electrification:
- Innovation and trials to reduce costs to increase uptake of low temperature heat electrification technologies.
- Innovation to develop technologies for medium and high temperature (>650°C) heat applications by 2030.
- Smart technologies, storage and demand side response to provide flexibility and allow industry to use energy when it is cheapest and cleanest.
For bioenergy combined with CCUS (BECCS): further research to understand how to increase supplies of sustainable biomass and ensure it is used in the most impactful way.
Innovation to unlock other low carbon fuels including waste, ammonia and process heat from sources such as advanced nuclear reactors and alternative technology options, such as large-scale hydrogen fuel cells.
Research to understand the best fuel switching options for a range of sectors. Zero emission options for Industrial Non-Road Mobile Machinery.
Capturing and storing industrial emissions
(Research and innovation needs relating to CCUS generally are covered in section 4.3. This table covers needs relating specifically to industry).
The maturity of CCUS technology varies across industrial applications. CCUS applied to sources such as steel and cement is still in the demonstration or prototype stage and requires innovation in the near term.
- Heat recovery solutions and integration of the capture process with the wider site.
- First-of-a-kind CCUS demonstration plants across industrial sources.
- Understanding of how capture rates can be improved, particularly for flue gas streams with low CO2 concentration.
- Research into bespoke solutions, tailored to the specific conditions on site including treatment of flue gas impurities.
- Dispersed sites may not easily connect to CO2 transport and storage infrastructure. Innovation in alternative means of transport and possibly capture technology is required, such as modular carbon capture.
- Understanding the potential for local synergies between carbon capture and carbon utilisation opportunities, such as producing synthetic fuels or other chemicals.
Figure 13: Industry research and innovation needs timeline
Improving resource and energy efficiency:
- Reduce use of raw materials and development of alternative, renewable feedstocks, including in chemicals and cement production - medium term to longer term (2025 to 2030s and beyond)
- Advanced technologies and new manufacturing processes, such as new steelmaking technologies to produce ‘green steel’ - medium term to longer term (2025 to 2030s and beyond)
- Research and testing related to new heat recovery techniques - short to longer term (2020 to 2030s and beyond)
- Advanced manufacturing technologies to create lighter, cheaper and less resource intensive materials - short to medium term (2020 to 2030)
- Digital Twin technology to identify potential issues and inefficiencies and improve the cost effectiveness, efficiency and flexibility of production - short to medium term (2020 to 2030)
- Reduce the impact of a product in use, including light weighting, eco-design for disassembly, remanufacture or recycling of end-of-life parts or products, or products made with reused/recycled and alternative materials, increasing product lifetime or re-use - short to medium term (2020 to 2030)
- Business model innovation (leasing, product service systems or pay as you go) that supports more efficient use of resources - short to medium term (2020 to 2030)
- End of life: Advanced separation and sorting of waste that enables more and higher quality recycling; design and produce biodegradable and compostable materials with properties comparable to difficult to recycle materials such as engineering plastics - medium term to longer term (2025 to 2030s and beyond)
Switching to low and zero-carbon fuels and feedstocks (such as electricity, hydrogen and biomass):
- Hydrogen: Demonstrate low carbon hydrogen as a feedstock for industrial sectors, new products and synfuels - short to medium term (2020 to 2030)
- Hydrogen: Technologies for equipment categories with potential for significant demand for hydrogen, including industrial boilers and combined heat and power systems - short to medium term (2020 to 2030)
- Hydrogen: Technologies for high temperature direct firing, for example, primary steel production, glass, ceramics, chemicals - short to medium term (2020 to 2030)
- Hydrogen: Innovation to connect dispersed sites to hydrogen networks in industrial clusters - medium term to longer term (2025 to 2030s and beyond)
- Hydrogen: Making existing gas equipment ‘low carbon ready’ - short to medium term (2020 to 2030)
- Hydrogen: Innovation to reduce costs to support hydrogen being competitively priced - short to medium term (2020 to 2030)
- Electrification: Innovation and trials to reduce costs to increase uptake of market-ready low temperature heat electrification technologies - short to medium term (2020 to 2030)
- Electrification: Develop technologies for medium and high temperature (>650°C) heat applications - short to medium term (2020 to 2030)
- Electrification: Smart technologies, storage and demand‑side response to provide flexibility and allow industry to use energy when it is cheapest and cleanest - short to medium term (2020 to 2030)
- Bioenergy with CCUS (BECCS) - further research on how limited supply sustainable biomass should be used and how supply could be increased - short to medium term (2020 to 2030)
- Innovation to unlock other low carbon fuels including waste, ammonia and process heat from sources such as advanced nuclear reactors and large scale hydrogen fuel cells - short to medium term (2020 to 2030)
- Research and understand the best fuel switching options for a range of sectors with different characteristics, where the optimal pathway to net zero is uncertain - short term (2020 to 2025)
- Zero emission options for Industrial Non-Road Mobile Machinery - short to medium term (2020 to 2030)
Capturing and storing industrial emissions:
- Heat recovery solutions and integration of the capture process with the wider site - short to medium term (2020 to 2030)
- First of a kind CCUS demonstration plants across industrial sources - short to medium term (2020 to 2030)
- Improve capture rates, particularly for flue gas streams with low CO2 concentration - medium term to longer term (2025 to 2030s and beyond)
- Research into bespoke, site specific CCUS solutions including treatment of flue gas impurities - medium term to longer term (2025 to 2030s and beyond)
- Innovation for alternative means of transport and capture for dispersed sites that may not easily connect to transport and storage infrastructure - medium term to longer term (2025 to 2030s and beyond)
- Research the potential for local synergies between carbon capture and carbon utilisation opportunities - medium term to longer term (2025 to 2030s and beyond)
Policy ambitions:
- Connecting 2 of the UK’s major industrial clusters to decarbonisation infrastructure - by 2025 and 4 by 2030
- 20 TWh of fossil fuels replaced with low carbon alternatives by 2030, and 6 Mt CO2e captured by 2030 and 9 Mt CO2e - by 2035
- Industrial emissions to fall by two-thirds by 2035 compared to todays’ levels and by 72% for sixth carbon budget - by 2035
- World’s first net zero industrial cluster - by 2040
- No fossil fuel in use (unless carbon emissions are captured) - by 2050
4.2.2 Scaling up the supply and demand for low carbon hydrogen
Context
Hydrogen is one of a handful of new, low carbon solutions that will be critical for the UK’s transition to net zero. As part of a deeply decarbonised, highly renewable energy system, low carbon hydrogen could be a versatile replacement for high-carbon fuels used today. The UK is therefore expected to need significant amounts of low carbon hydrogen in the energy system[footnote 25]. Due to its flexibility, hydrogen could be used in buildings for heating and cooking, power and transport sectors and as a fuel or feedstock for industrial processes and products. It is a leading option where electrification is difficult, such as for high temperature heat in industry (like kilns) and for heavy transport including some shipping, aviation and buses as well as HGVs and trains. It could also provide energy system benefits, including for managing peak loads and grid balancing.
The Hydrogen Strategy[footnote 26] sets out a roadmap for how we will meet our ambition of working with industry to deliver 5GW of low carbon hydrogen production capacity by 2030, along with the development of associated infrastructure, supply chains and hydrogen end-uses across different sectors. Over the 2020s, government will support the creation of hubs where low carbon electricity, CCUS and hydrogen congregate. The Hydrogen Advisory Council has been created to improve coordination with industry to overcome near-term challenges.
Beyond our 2030 ambition, hydrogen demand is expected to increase rapidly over the 2030s and 2040s, and analysis suggests we could need 7 – 20 GW of low carbon hydrogen production capacity in 2035[footnote 27]. This scale-up underlines the need for innovation support to bring forward a greater range of high efficiency, novel technologies across the hydrogen value chain.
Currently, there are 2 main focuses for low carbon hydrogen production:
- CCS enabled hydrogen: through reformation of natural gas using steam methane reformers or autothermal reformers, coupled with CCS. Due to CO2 capture rates, there will be some residual emissions with these technologies. GGR technologies (including DACs) will be needed to make up this deficit.
- Electrolytic hydrogen: through electrolysis of water using low carbon electricity. The main electrolyser technologies are alkaline electrolysis, proton and anion exchange membrane electrolysis and solid oxide electrolysis cells. If produced using renewable electricity, this production method is zero carbon.
However, there are also other potential hydrogen production routes which are the subject of active research and development. These include using nuclear energy for high temperature processes, biomass gasification with CCUS (‘biohydrogen’), photocatalysis and solar thermochemical production. Research and innovation on these production routes can help accelerate the evolution of the hydrogen economy in the UK and support critical decisions on hydrogen’s role.
Production and storage of hydrogen may also play an important role in smoothing the intermittency of renewable energy. Hydrogen has the potential to be stored and used when demand requires it to support security of supply and to meet peaking loads. We will need to see significant development and scale-up of hydrogen network and storage infrastructure over the 2020s if low carbon hydrogen is to play its role in supporting UK decarbonisation[footnote 28]. Various options exist for storing hydrogen at scale, including as a compressed gas (such as in underground salt caverns), using another chemical carrier such as ammonia or through the development and use of solid hydrogen storage systems such as solid phase absorbent technology.
Hydrogen distribution will also need to be developed. Hydrogen can be transported by road, rail or boat as a compressed gas or liquid, or using a different storage medium, such as ammonia. For larger scale transmission and distribution, pipelines can be used. Newer natural gas pipeline networks could be used to distribute hydrogen through blending (meaning hydrogen injection into gas grids) with the potential to convert them entirely to hydrogen over time.
International collaboration on hydrogen innovation is crucial to accelerate cost reductions. As a co-lead of the Mission Innovation (MI) Clean Hydrogen Mission[footnote 29], the UK is working with other MI members and the private sector to catalyse cost reductions in low carbon hydrogen across the value chain, with the goal to increase the cost-competitiveness of low carbon hydrogen by reducing end-to-end costs to $2 per kilogram by 2030. In addition, working with partner initiatives and organisations, the Mission members will deliver at least 100 large-scale integrated clean hydrogen valleys worldwide by 2030. These will be demonstration projects to test different production, storage and transport methods and end-use applications, aiming to reach critical levels of investment and experience.
Figure 14: System interlinkages between Hydrogen and other sectors
Description of figure 14
Figure representing the system interlinkages between Hydrogen and the 6 other sectors:
Natural resources, Waste and F-gases -
- H2-BECCS (hydrogen production from biomass using CCUS)
- Water demand for hydrogen production
Transport -
- Hydrogen as fuel for surface transport, aviation and shipping
- Co-located hydrogen production and transport refuelling hubs
Heat and buildings -
- Hydrogen for buildings heat
- Hydrogen and hybrid- hydrogen appliances
Industry -
- Hydrogen as fuels for industrial processes
- Hydrogen production and storage co-located with industrial clusters
Power -
- Hydrogen production via electrolysis
- Power generation from hydrogen (such as peaking)
- Hydrogen appliances and vehicles
CCUS & GGRs -
- Hydrogen production via methane reforming with CCUS
Research and innovation: challenges and needs
Demonstrating low carbon hydrogen production methods as efficient, reliable and low-cost at increasing scales is a key innovation priority for the next 5 - 10 years. A number of UK projects are already planned for both methane reformation with CCUS and electrolytic hydrogen routes, but ongoing innovation is required alongside a better understanding of the wider system and environmental impacts.
Hydrogen end-uses are covered in other sections of the report (such as industry, buildings, power and transport).
Efficient, cost-effective production of low carbon hydrogen at scale
Demonstrate hydrogen production using next generation methane reforming technology, coupled with CCUS.
Demonstrate hydrogen production via electrolysis, initially at the 10s MW scale at different sites. Also, continue to develop next generation electrolysers and demonstrate advanced manufacturing methods to drive costs and efficiency.
Continue to improve efficiency and reduce costs of production as deployment increases, including a range of high efficiency / novel technologies across the value chain.
Research and development to further drive down emissions from hydrogen production. Develop lower TRL and novel production technologies (for example, hydrogen from next generation nuclear and biohydrogen with CCUS) to enable demonstration in the 2020s or 2030s.
Alternative ammonia production processes, to improve or replace high energy intensive Haber-Bosch process.
Demonstrating effective, low-cost methods of bulk hydrogen transportation and storage
Transportation:
Build the evidence base for injecting hydrogen into the existing gas grid, both the safe blending of up to 20% hydrogen and full conversion to 100% hydrogen.
Research and innovation to improve efficiency and costs of hydrogen distribution.
Understand and model the needs of hydrogen grid transmission.
Progress technologies and infrastructure for international transport (for example, port infrastructure, liquefaction technologies, hydrogen carriers and associate conversion technologies).
Progress techniques, technologies, and materials required to compress hydrogen, including for storage and transport.
Develop infrastructure and technologies for international transport and improve integration of different hydrogen networks.
Demonstrate longer distance hydrogen transmission – scaling-up to international transport in 2030s.
Develop components for safe hydrogen distribution and use. R&D to understand and address the safety challenges of storage and supply. Understand impact of hydrogen on distribution and storage materials.
Storage:
Enable efficient use of short and long-term storage options, including:
- Assess the impact of hydrogen on materials for storage and supply and identify options / solutions.
- Identifying the optimal storage solutions across the hydrogen system, including developing novel short term, transportable and large-scale solutions.
- Enable efficient use of short- and longer-term storage options, including assessing the need for underground storage.
- Develop options for hydrogen storage including medium scale storage, increasing the efficiency of ammonia storage and cracking, and solid hydrogen storage systems such as solid phase absorbent technology.
Power generation
Explore the role of fuel cells in applications such as decentralised power, industrial CHP and heat networks.
Develop and demonstrate large, centralised hydrogen fuelled power generation options, such as hydrogen turbines, in the 2020s.
Effective use of hydrogen at the system level
Whole systems analysis to understand systems level questions, such as interfaces within and between hydrogen sub-sectors, interactions with broader energy system, trade-offs between technologies and uses, and optimal deployment scenarios.
Research and innovation to align hydrogen production and demand, enable supply chain development and improve safe integration into the wider energy system.
Understanding how hydrogen will impact the environment and society
Assess the atmospheric and terrestrial environmental impacts of a hydrogen economy. As the hydrogen economy develops and is deployed, develop mitigation measures against any negative environmental impacts, including water usage.
Understand and forecast impact of hydrogen on international markets.
Ongoing research into consumer attitudes / acceptance towards hydrogen and identify measures to build consumer confidence.
Figure 15: Hydrogen research and innovation needs timeline
Efficient, cost effective production of low carbon hydrogen at scale:
- Demonstrate hydrogen production using next generation methane reforming technology coupled with CCUS - short to medium term (2020 to 2030)
- Demonstrate hydrogen production via electrolysis, initially 10s MW scale at different sites & develop next generation electrolysers / advanced manufacturing methods to drive costs and efficiency - short to medium term (2020 to 2030)
- Increase efficiency and reduce costs of production technology and processes as deployment increases - longer term (2025 to 2030s and beyond)
- Research and development to further drive down emissions from hydrogen production
- Development of lower TRL and novel production technologies (for example, hydrogen from next generation nuclear / biohydrogen with CCUS to enable demonstration) - short to longer term (2020 to 2030s and beyond)
- Alternative ammonia production processes, to improve or replace highly energy intensive Haber-Bosch process - medium to longer term (2025 to 2030s and beyond)
Demonstrating effective, low-cost methods of bulk hydrogen transportation and storage:
- Build evidence for injecting hydrogen into the existing gas grid - short term (2020 to 2025)
- Research and innovation to improve the efficiency and costs of hydrogen distribution - medium term (2025 to 2030)
- Understand and model the needs of hydrogen grid transmission - short to medium term (2020 to 2030)
- Develop infrastructure and technologies for international transport and improve integration of different hydrogen networks - short to medium term (2023 to 2030)
- Demonstrate longer distance hydrogen transmission - scaling up to international transport in 2030s - medium to longer term (2027 to 2030s and beyond)
- Assess the impact of hydrogen on materials for storage and supply and identify options / solutions - short term (2020 to 2025)
- Identify the optimal storage solutions across the hydrogen system, including developing novel short term, transportable and large-scale solutions - short term (2020 to 2025)
- Enable efficient use of short and longer term storage options, including assessing the need for underground storage - short to medium term (2020 to 2030)
- Develop options for hydrogen storage including medium scale storage, increasing efficiency of ammonia storage and cracking - short to medium term (2020 to 2030)
- Develop solid hydrogen storage systems such as solid phase absorbent technology - short to medium term (2020 to 2030)
Power generation:
- Explore the role of stationary fuel cells in applications such as decentralised power, industrial combined heat and power and heat networks - short to medium term (2020 to 2030)
- Develop and demonstrate large centralised hydrogen fuelled power generation options, such as hydrogen turbines, in the 2020s - short to medium term (2020 to 2030)
Effective use of hydrogen at the system level:
- Analysis of hydrogen at the whole system level - short term (2020 to 2025)
- Enable ecosystems for hydrogen research and innovation - short to medium term (2020 to 2030)
- Align production and demand - short to medium term (2020 to 2030)
- Address safety questions and incorporate safety by design into hydrogen technologies - short to longer term (2020 to 2030s and beyond)
- Research and innovation to enable supply chain development across the value chain and address technology barriers - short to longer term (2020 to 2030s and beyond)
- Research and innovation to optimise integration into the electricity / energy system - short to longer term (2020 to 2030s and beyond)
Understanding how hydrogen will impact the environment and society:
- Assess environmental impact of a hydrogen economy (by 2025) and mitigate against impacts (on-going) - short term (2020 to 2025)
- Address new questions as technologies are developed and deployed - short to longer term (2020 to 2030s and beyond)
- Understand and forecast impact of hydrogen on international markets - short to medium term (2020 to 2030)
- Understand and address acceptance barriers - short to medium term (2023 to 2030)
Policy ambitions:
- Complete hydrogen neighbourhood trial and 20% blending testing - 2023
- Ambition for 1GW hydrogen production capacity - 2025
- Hydrogen village trial (2025); Potential hydrogen town - by end of 2020s
- 5GW low carbon production capacity - by 2030
- 1 Net zero carbon industrial cluster - 2040
4.3 Carbon Capture Utilisation and Storage (CCUS) and Greenhouse Gas Removal (GGR)
Context
CCUS technologies are likely to have applications across many parts of the economy as the UK transitions to net zero. Tata Chemicals CCU plant is set to be the UK’s first operational project. In the near term, the government’s ambition is for CCUS to be deployed in 2 industrial clusters by the mid-2020s and 4 low-carbon hubs, spread across the UK, capturing 10 MtCO2e annually by 2030[footnote 30]. This will demonstrate CCUS technology across multiple industrial sectors, providing crucial ‘learning-by-doing’ for industry, academia and the wider UK supply chain, and is key to confidently scaling CCUS in the 2030s.
In addition, CCUS infrastructure is needed to support GGR solutions. Even in ambitious decarbonisation scenarios there are likely to be some residual GHG emissions in 2050. The Net Zero Strategy estimates that 75-81 MtCO2e of engineered removals will be needed per year by 2050 to compensate for residual emissions in the most difficult to decarbonise sectors such as aviation and parts of agriculture and industry[footnote 31].
CCUS technology will be applied to larger point sources of CO2 emissions, including stack emissions or process emissions. Potential applications include:
- Industry. CCUS has the potential to be applied to a variety of industry sectors and processes. This includes emissions captured from the combustion of fossil fuels in industrial processes as well as non-combustion emissions such as those from limestone calcination in cement production.
- Bioenergy with CCUS. Combining bioenergy production with CCUS technologies (BECCS) offers one of the main routes to removing CO2 from the atmosphere, providing that sustainable biomass feedstocks are used. BECCS could potentially be applied in many sectors including power generation, hydrogen production, renewable fuel production (such as sustainable aviation fuel) and industry.
- Gas power with CCUS. This has the potential to enable continued fossil fuel combustion for electricity generation whilst substantially reducing emissions. This offers a potential route to low carbon baseload and / or dispatchable generation.
- Hydrogen production with CCUS. Applying CCUS technology to methane reformation provides a way of producing low carbon hydrogen from natural gas or biomass. This is likely to be a particularly important route providing the bulk supply of low carbon hydrogen necessary to establish the UK hydrogen economy in the 2020s, while electrolytic hydrogen technologies scale-up and costs come down.
Innovation is needed to improve current CCUS technology and to deploy next-generation technology. Next-generation CCUS technology aims to have better performance, lower environmental impact and be more widely applicable than current technology.
GGR solutions typically fall into 2 broad categories and it is likely a combination of several approaches will be necessary to achieve the level of removals required for net zero.
Engineering-based solutions include:
- Bioenergy with Carbon Capture and Storage (BECCS)
- Biochar - a charcoal-like product produced by the combustion of biomass in the absence of oxygen (known as pyrolysis). This may be added to soils to increase carbon storage in soil.
- Direct air carbon capture and storage (DACCS or DAC) – the use of chemical processes to capture CO2 and other GHGs directly from the atmosphere and permanently store it in geological formations or use it to generate carbon containing fuels.
- Enhanced weathering - involves spreading crushed minerals over the land surface to increase chemical reactions which remove CO2 from the air.
- Sea water CO2 (and other GHG) removal solutions.
Nature-based solutions (covered in section 4.6) include:
- Afforestation - increasing forest area to raise CO2 absorption.
- Habitat restoration - the rewetting and restoration of peatlands, wetlands and other coastal habitats, which in turn enhances natural carbon absorption from the atmosphere.
Monitoring, reporting, and verification (MRV) of emissions removals will be key for these technologies to reach commercial scales. For some GGR approaches, the amount of carbon captured and stored can be easily measured and may not require periodic monitoring. In others, establishing this with necessary certainty and verifying that carbon remains secure will be more challenging. Both biological storage (such as soil or trees) and geological storage (such as sub-surface geological formations) are recognised as potential pathways, but they vary significantly in terms of durability of storage and associated risk of reversal.
CCUS has potential to be a significant opportunity for the UK, combining expertise on offshore engineering and sub-surface geology and large reservoir space for CO2 storage close to major European generators. The UK also has an excellent academic pedigree in early-stage scientific research and is well placed to develop a global leadership position in GGR technologies. The upcoming Biomass Strategy, due in 2022, will further consider the role of BECCS in delivering negative emissions and we will consult on business models for engineered GGRs in Spring 2022. The UK is well placed to develop these strengths and create significant export and growth opportunities as domestic and international supply chains develop, including options for CO2 use in the manufacture of synthetic fuels and other chemicals and products, with the International Energy Agency suggesting that all synthetic fuels produced post-2040 will be either of biomass or DAC origin[footnote 32].
Figure 16: System interlinkages between CCUS &GGRs and other sectors
Description of figure 16
Figure representing the system interlinkages between CCUS & GGRs and the 6 other sectors:
Natural resources, Waste and F-gases -
- Afforestation, soil carbon, peatland restoration
- Capture of GHGs from landfill and other waste processes
Transport -
- CCUS to balance residual transport emissions, such as long-haul aviation
Heat and buildings -
- Biogenic building materials or other built environment carbon storage opportunities
Industry -
- CCUS applied to industrial processes
- CO2 transport and storage infrastructure focussed on industrial clusters
Power -
- Power generation via BECCS and gas with CCUS
- Power demand from DACs
Hydrogen -
- Hydrogen production via methane reforming with CCUS
Research and innovation: challenges and needs
CCUS, BECCS and DACs technologies will ultimately rely on much of the same supporting infrastructure and will face similar challenges in terms of business models, financing and risk sharing arrangements, and policy and regulatory requirements. The table below covers research and innovation needs common to these technologies. Research and innovation needs related to nature-based removals and sustainable biomass feedstock production, specifically perennial energy crops and short rotation forestry, are covered in section 4.6.3 in the context of the management of natural resources.
Capturing CO2 from point sources, efficiently and at low-cost
Pre-combustion capture – gas: advanced reformer technologies to unlock the potential to combine hydrogen production with CCS for power. Cost reduction is possible using cheaper and more energy-efficient materials and processes.
Post-combustion capture – gas & solid fuels: R&D into new solvent and adsorption processes to reduce costs and improve capture performance as well as reduce regeneration costs, corrosion effects, environmental impact, and product degradation.
Oxy-combustion: new technologies for lower-cost air separation in oxy-combustion.
Other advanced low-cost capture technologies such as calcium looping.
Reduce the parasitic load of capture technologies to improve efficiency.
Increase CO2 capture rates and efficiency of carbon capture technologies.
Research, development and demonstration related to CCUS and GGR supply chain development and plant operations.
Removing GHGs directly from the air or sea, efficiently and at low-cost
RD&D for a range of options for capturing CO2 and other GHGs directly fromthe air or sea:
- Higher TRL Direct Air Capture (DAC) technologies as well asapproaches such as enhanced weathering of silicate rocks.
- Novel (lower TRL) approaches for capturing CO2 orother GHGs such as methane and N2O.
Innovations in monitoring and verification to help verify estimates of CO2captured where it cannot be measured by volume.
Reducing energy demand from engineered removal technologies
Improve efficiency and reduce energy demand of engineered GGR technologies,for example, DACs requires electricity to run fans to move air through or across a capture medium and heat to separate captured CO2 from reactants.
Exploring routes to deploy BECCS
(See also section 4.1.4, 4.2.1 and 4.6.3).
Develop and demonstrate efficient, cost-effective biomass gasification technologies that can produce high quality syngas and be co-developed with BECCS deployment (hydrogen and renewable fuel production).
Explore routes to innovate and optimise BECCS supply chains to combine BECCS with other existing and emerging technologies (combustion, gasification, anaerobic digestion, etc.) to produce power, heat, sustainable transport fuels (such as sustainable aviation fuel), or hydrogen, while fully assessing the environmental consequences of these approaches.
CO2 transport and storage infrastructure
CO2 Transport:
- Geographical cluster development to enable the identification of lowest-cost infrastructure opportunities.
- Research into sharing of infrastructure and capacity for transport and CO2 storage.
CO2 Storage:
- De-risking and developing solutions for the scale-up of both short and long-term CO2 stores, including technologies that characterise the connectivity of sands and structural features and innovation to tackle challenges that arise from the build out of planned full-scale stores.
- Technologies and methods that model, simulate, appraise and monitor stores faster, and with a higher degree of confidence. To include modelling of stores at broad geographical scales and to support optimisation, planning and management of stores in real-time.
- Measuring, Monitoring, and Verification of CO2 storage to prevent, observe, and report leaks. Improve long-term monitoring techniques, move towards performance-based standards.
- Development of depleted oil and gas reservoir storage technologies and methodologies to enable offshore CO2 storage.
- Unlocking the potential of CCUS on more dispersed sites that cannot easily connect to CO2 transport and storage infrastructure, innovation in alternative means of transport and storage and possibly capture technology is required, such as ship transport (port-to-offshore) and land-based gas transport (rail) solutions.
Developing economic ways to utilise captured CO2 in products or processes
Development of carbon utilisation opportunities, for example, sequestering carbon in long-life products or in the production of synthetic fuels and other chemicals - acknowledging the need to better understand lifecycle emissions and future innovation needs.
Research to deepen understanding of the role of modern methods of construction on net zero.
Creating the conditions for future scale-up and deployment / commercialisation
Scaling-up CCUS technologies and GGR approaches from pilot to commercial first-of-a-kind demonstrations needs to happen over the next 10–15 years to allow widespread roll-out from the 2030s onwards. This implies:
- Demonstrating CCUS at scale across a range of industrial applications.
- Demonstrating hydrogen production using next generation methane reforming technology with CCUS.
- Demonstrating BECCS and gas CCS at scale in the power sector.
- Demonstrating DACs and other GGR approaches at scale.
Policy, regulatory and risk-sharing frameworks and commercially acceptable business models will be as important as technology innovation in enabling the widespread deployment of CCUS and GGR. Further RD&D may be needed to develop and test these frameworks and business models, building on work currently being undertaken by BEIS, including solutions for the integration and interlinking of CCUS technologies to achieve combined capture, utilisation and storage.
Research into public attitudes towards CCUS and GGR and how this could be mobilised to support the required deployment of these technologies.
Monitoring, Reporting, and Verification
Monitoring, reporting and verification methodologies for different GGR approaches must be developed by 2030 to ensure commercial-scale roll-out can occur.
Before GGRs can be fully deployed on a commercial basis, in either voluntary markets or as part of a compliance-based approach, it will be vital to understand:
- How much CO2 has been removed from the atmosphere.
- When that removal has taken place.
- At what rate that removal will persist, and for how long.
- In what type of sink it has been stored.
- The characterisation and durability of that store.
- The point at which a given store reaches maximum stability / saturation.
To ensure the credibility of a removal, and support market legitimacy and perception, it is also important to consider:
- Additionality – proving the removal activity is additional to what was happening anyway, in the absence of the GGR intervention.
- Avoid double counting – ensuring geographical accountability and accuracy, so that a removal is not credited or accounted for twice.
Managing environmental impacts and co-benefits
Further understand how GGR / CCUS technologies impact the environment.
Figure 17: CCUS and GGR research and innovation needs timeline
Capturing CO2 from point sources, efficiently and at low cost:
- Pre-combustion capture – gas: advanced reformer technologies & cheaper and more energy-efficient materials to reduce cost - short to medium term (2020 to 2030)
- Post-combustion capture – gas & solid fuels: Research and development into new solvent and adsorption processes to lower cost and improve capture performance - short term (2020 to 2025)
- Oxy-combustion: New technologies for lower-cost air separation in oxy-combustion - short to longer term (2020 to 2030s and beyond)
- Other advanced low-cost capture technologies such as calcium looping - medium to longer term (2025 to 2030s and beyond)
- Reduce the parasitic load of capture technologies to improve efficiency - short to medium term (2020 to 2030)
- Increase CO2 capture rates and efficiency of carbon capture technologies - short to medium term (2020 to 2030)
- Research, development and demonstration related to CCUS and GGR supply chain development and plant operations - short to medium term (2020 to 2030)
Removing GHGs directly from the air or sea, efficiently and at low cost:
- Research, development and demonstration for a range of options capturing CO2 and other GHGs directly from the air or sea across a range of TRL levels - short to longer term (2020 to 2030s and beyond)
- Monitor and verify improvements to help verify estimates of CO2 captured - medium to longer term (2025 to 2030s and beyond)
Reducing energy demand from engineered removal technologies:
- Improve efficiency and reduce energy demand of engineered GGR technologies - medium to longer term (2025 to 2030s and beyond)
Exploring routes to deploy BECCS:
- Develop and demonstrate efficient, cost-effective biomass gasification technologies - short to medium term (2020 to 2030)
- Optimise biomass BECCS supply chains to combine BECCS with other technologies (combustion, gasification, anaerobic digestion, etc) to produce power, heat, sustainable transport fuels, or hydrogen, while fully assessing the environmental consequences - short to medium term (2020 to 2030)
CO2 transport and storage infrastructure:
- Develop geographical cluster to enable the identification of lowest-cost infrastructure opportunities - short to medium term (2020 to 2030)
- Research into sharing of infrastructure and capacity for transport and CO2 storage - short to medium term (2020 to 2030)
- De-risking and developing solutions for the scale-up of both short and long-term CO2 stores - short to medium term (2020 to 2030)
- Methods that model, simulate, appraise and monitor stores faster, and with a higher degree of confidence - medium to longer term (2025 to 2030s and beyond)
- Measuring, Monitoring, and Verification of CO2 storage to prevent, observe, and report leaks - medium to longer term (2025 to 2030s and beyond)
- Development of depleted oil and gas reservoir storage technologies to enable offshore CO2 storage - medium to longer term (2025 to 2030s and beyond)
- Innovation in alternative means of transport, storage, and capture of CO2 for dispersed sites including sharing of infrastructure / capacity - longer term (2030s and beyond)
Developing economic ways to utilise captured CO2 in products or processes:
- Development of carbon utilisation opportunities, for example, sequestering carbon in long-life products. Also in the production of synthetic fuels and other chemicals - medium to longer term (2025 to 2030s and beyond)
- Research to deepen understanding of the role of modern methods of construction on net zero - short term (2020 to 2025)
Creating the conditions for future scale-up and deployment / commercialisation:
- Demonstrate CCUS at scale across a range of industrial applications - short to medium term (2020 to 2030)
- Demonstrate hydrogen production using next generation methane reforming technology with CCUS - short to medium term (2020 to 2030)
- Demonstrate BECCS and gas CCS at scale in the power sector - medium to longer term (2025 to 2030s and beyond)
- Demonstrate DACCS and other GGR approaches at scale - longer term (2030s and beyond)
- Develop and test policy, regulatory and risk-sharing frameworks and commercially acceptable business models - short to medium term (2020 to 2030)
- Research into public attitudes towards CCUS and GGR technologies - short to medium term (2020 to 2030)
Monitoring, Reporting and Verification (MRV):
- Development of technology-specific MRV to enable robust carbon accounting and assignment of credits to support carbon markets - short to medium term (2020 to 2030)
Managing environmental impacts and co-benefits:
- Further understand how CCUS / GGR technologies impact on the environment - short to medium term (2020 to 2030)
Policy ambitions:
- Connect the UK’s major industrial clusters to decarbonisation infrastructure - 2 by 2025 and 4 by 2030
- 3 MtCO2e captured - by 2030
- For GGRs, ambition for 5MtCO2 of engineered removals annually - by 2030
- World’s first net zero industrial cluster - by 2040
- No fossil fuel in use (unless carbon emissions are captured) - by 2050
4.4 Heat and buildings
Context
The UK has around 30 million buildings[footnote 33] which are collectively responsible for around 30% of total UK GHG emissions (direct and indirect)[footnote 34]. Most buildings currently rely on natural gas for heating, hot water and cooking, whilst others use electricity, oil, liquified petroleum gas (LPG) or solid fuels. Much of the UK’s existing building stock also has poor levels of energy efficiency; for example, approximately two-thirds of UK homes have an EPC rating of D or below[footnote 35], meaning that much of the heat generated is wasted. To reach net zero, virtually all heat in buildings must be decarbonised by 2050.
From 2025, the Future Homes Standard will ensure that all new-build homes will have low carbon heating and high levels of efficiency, meaning that no further energy efficiency retrofit work will be necessary to enable them to become zero-carbon over time as the electricity grid continues to decarbonise.
For net zero, the construction industry will also need to adopt low carbon materials, low carbon construction techniques, repurpose existing buildings where practical and improve the efficiency of its supply chain operations (including maximising the potential of local or regional manufacturing).
Alongside this, a key challenge is to retrofit the significant proportion of homes and non-residential buildings[footnote 36] that require remedial work to achieve high standards of energy efficiency and to use low carbon heating sources. The diverse nature of the UK’s building stock, which includes large commercial properties, public sector buildings (such as schools and hospitals), and a range of domestic properties (from flats to large, detached homes), also means that decarbonisation measures will be informed by local and site-specific requirements, as well as end-user preferences and needs. Developing appealing solutions and an understanding of end-user choices are both critical, as decarbonising buildings may cause disruption for homes and workplaces.
For decarbonising heat there are various potential options, including heat pumps, hydrogen, heat networks and biomethane. Some of these solutions may also have a role to play in delivering cooling, particularly as the UK adapts to the impacts of rising global temperatures. The government has committed to making a strategic decision by 2026 on the long-term role of hydrogen for heating, including through community hydrogen heating trials, and to increase the number of heat pumps installed to 600,000 per year by 2028. Innovation will be critical to ensure the right solutions are available and cost-effective. We will also need innovation in business models, finance and across supply chains.
Future heating systems are also likely to be increasingly integrated with other forms of energy production, storage and demand, at both building and community scales. End-user preferences may become more specific, for example wanting more control over heating systems, comfort cooling as well as heating, and more engagement with energy consumption and tariffs. These changes point to an increasing need for smart technologies, behavioural insights and new business models and market arrangements. Buildings will be increasingly linked to the power and transport sectors and associated infrastructure, with interlocking innovation needs for integrated, low carbon, flexible technologies installed in homes and businesses across the country. We need to understand and prepare for the energy requirements of a decarbonised building stock, whilst maximising the potential to enable system-wide flexibility.
Improved energy efficiency and flexible demand will also help to tackle fuel poverty for domestic end-users, improve wellbeing, and help to reduce energy costs for both domestic and non-domestic end-users.
Retrofitting millions of buildings will create hundreds of thousands of UK jobs[footnote 37] and the creation of a domestic market for low carbon materials and products will help drive the transformation of UK industry, in turn supporting exports and growth. Heat network engineering, procurement, construction management and automatic monitoring services are also projected to expand along with potential new markets in hydrogen heating for buildings. Energy efficiency measures could support up to £6 billion gross value added (GVA) and 175,000 jobs by 2030[footnote 38]. The development of and integration into domestic and international supply chains by UK companies will be essential to secure maximum economic benefit and market share.
Figure 18: System interlinkages between Heat & Buildings and other sectors
Description of figure 18
Figure representing the system interlinkages between Heat and buildings and the 6 other sectors:
Natural resources, Waste and F-gases -
- Bioenergy for hard- to-decarbonise buildings
- Biogas grid injection
- Biogenic building materials
Transport -
- Electric vehicle charging
- Smart home technologies
- Demand side response for grid balancing
- Low-carbon transport of materials
Industry -
- Possibility of shared hydrogen infrastructure
- Supply of low carbon building materials
Hydrogen -
- Hydrogen for buildings heat
- Hydrogen and hybrid- hydrogen appliances
Power -
- Electrification of heat (heat pumps and heat networks)
- Smart home technologies & appliances
- Demand side response for grid balancing
CCUS & GGRs -
- Biogenic building materials or other built environment carbon storage opportunities
Research and Innovation: challenges and needs
Given the diversity of potential heat decarbonisation options and the varied contexts in which these will be applied, research and innovation over the next decade will need to develop and test solutions at increasing scale in a range of different situations. For hydrogen, this involves supporting industry to deliver a hydrogen neighbourhood trial by 2023, a hydrogen village trial by 2025, with an aim for a hydrogen town by 2030. For heat pumps, optimising the installation process, in particular driving down installation costs and product innovation, are the immediate priorities.
Other priorities include accelerating delivery of decarbonisation solutions through innovative business models and offers, such as an integrated Whole House Retrofit approach, and research to understand how end-users may want to access solutions that best suit their needs. Developing lower-cost, lower-disruption solutions for hard-to-treat properties, such as solid wall insulation, is another key priority. In parallel, research and innovation in the construction industry is needed to transform the design, construction and materials used in buildings.
Develop an enabling environment for system-wide decarbonisation of building stock
Leverage data to research and establish the real energy performance of buildings to inform decisions and drive improvements.
Integrate retrofit solutions to identify and deliver the optimal mix of building fabric interventions and heating, cooling and storage technology, suited for different buildings and end-users.
Develop innovative business models (such as energy as a service, builders / installers who can offer a Whole House/Building Retrofit package rather than an individual measure) and market arrangements (such as time-of-use tariffs).
Further innovation to encourage standardisation of components, contracts, and installation practices to reduce costs and facilitate local supply chains.
Support organisations to develop and trial green finance options, including:
- Lender partnerships with energy efficiency and low carbon technology suppliers, stimulating development of a competitive green finance market.
- Incentivise home and business owners to make improvements.
Removing barriers to widespread energy efficiency retrofits in existing domestic and non-domestic buildings
Increase accessibility and availability of energy reduction and efficiency measures by encouraging innovation to:
- Reduce upfront cost through systems engineering, including testing whole building approaches (such as Whole House Retrofit) for domestic and non-domestic end-users.
- Reduce space requirements of retrofit and other energy efficiency solutions.
- Develop energy reduction and efficiency solutions for hard-to-treat properties as well as alternative market innovations to support energy efficiency.
- Develop the new supply chain models needed for deploying energy efficiency solutions, including whole house/building retrofit approaches, at scale.
- Develop low-cost and transparent ways of measuring real-world performance of energy efficiency measures (for example, though Smart Metering Enabled Thermal Efficiency Ratings) to incentivise improved performance and support new end-user services and products.
Developing, demonstrating, reducing the costs of and de-risking low carbon heating and cooling technologies
(Research and innovation needs relating to supply of clean hydrogen are covered in section 4.2.2).
For hydrogen:
- Carry out necessary research and innovation to demonstrate safety, feasibility and impacts of converting parts or the whole of the existing natural-gas network to hydrogen.
- Understand the wider implications of hydrogen as a fuel, for example, hydrogen emissions (upstream and downstream), and energy system impacts.
- Increase readiness and further innovation on hydrogen heating appliances and understand the impacts of safety measures on buildings, for example, hydrogen-ready boilers, particularly for commercial / industrial use, hybrid-hydrogen heat pumps, hobs / cookers.
- End user trials testing user acceptance involving practical demonstrations.
For heat pumps:
- Reduce costs and improve efficiency, for example, industrialised manufacture and supply chain innovation.
- Reduce disruption from installation and use of heat pumps, including time taken to install, space requirements, noise and ease of use.
- Develop solutions to enable the phase out high global warming potential refrigerants, including F-gases, and move towards the use of natural or low impact refrigerants (see also section 4.6.4).
- Trial innovative new business models to drive uptake, including flexibility services.
- Improve collection of performance data, including at low temperatures.
- Demonstrations of innovative heat pump solutions in a range of homes and at neighbourhood / district scale.
- Demonstrations of mass heat pump uptake in concentrated areas.
- Develop solutions that optimise heat pump deployment to provide wider system benefits, including demand shifting to utilise low carbon energy sources and to help manage local electricity network capacity.
For bioenergy in buildings:
- Better understand potential role of biomass and liquid biofuels for providing heat to off-gas grid properties, potentially with hybrid heat pump systems. Support needs to take into account wider environmental goals, such as the government’s statutory targets to improve air and water quality, and necessary innovation in abatement technologies for pollutant emissions from new fuels.
For anaerobic digestion:
- Routes to improve biomethane and / or hydrogen yield though the use of a variety of biomass feedstocks.
- Address the challenge of storing, spreading and, in some cases transporting digestate, in order to support identifying and growing new markets for digestate as a beneficial and sustainable product.
- Identify mitigation technologies and techniques best suited to address the potential negative impacts of digestate, including the impact of ammonia emissions on the government’s statutory air quality and biodiversity targets.
For gasification:
- Research and innovation into feedstocks and the gasification process, as described in 4.1.4, with heating in buildings a possible end-use for fuels from gasification.
Maximising the potential of heat networks
Research and trials to incentivise connections to heat and cooling networks, to minimise the need to compel or enforce connections in heat network zones.
Research and trials of disaggregated models of delivery or alternative business models to increase pace of delivery where zoning is an enabler and where it isn’t.
Research and trials of innovative solutions to access heat from existing and future heat sources; mine waste, geothermal, nuclear (AMR and SMR), industrial clusters, and Energy from Waste installations.
Research and trials to identify optimal ways of transitioning high temperature combined heat and power (CHP) systems to future net zero options.
Research and trials to understand the role of solar thermal in heat networks and the potential role of thermal stores for the wider system. See also section 4.1.1. Research to identify and develop innovative delivery models that enable effective expansion of networks in heat network zones over time.
Integrating smart, low carbon technologies and solutions
(Innovation needs for system flexibility are covered in section 4.1.1).
Develop and demonstrate standardised, cyber secure, interoperable smart systems to integrate low carbon technologies and energy efficiency at the building level, for example, heat-pumps (including hybrids), battery storage, hot water storage, electric vehicles, heating controls and smart appliances.
Innovation to enable technologies in buildings to provide appropriate data in appropriate formats according to energy data best practice to key parties such as networks, system operators, suppliers and service providers.
Improve understanding, modelling, and calculation methodologies for energy storage, for example how insulation, hot water tanks and batteries can and should be combined to provide an optimal solution.
Understanding end-user behaviour to drive uptake
(Further research and innovation needs related to end user behaviour and willingness and ability to flex demand is captured in section 4.1.1).
Improve understanding of barriers to deployment of energy efficiency improvements, smart technologies and low carbon heating, how this differs for different domestic and non-domestic end-user groups, and how it differs for different segments of the population, for example, factors such as age / life status, income, ownership status of the home, geographical factors, etc.
Develop understanding of the necessary advice and support needed to tackle low levels of awareness, empower end-users and support the necessary levels of uptake and acceptance for both near and longer-term deployment of low carbon heating and energy efficiency measures, including innovative financing trials.
Develop understanding of any impacts, of installing low carbon heating and energy efficiency measures on end-user usage habits.
Further research into end user green choices, acceptance and preferences to support near-term deployment ambitions.
Driving down emissions associated with construction
Development of new construction technologies, materials and techniques that can improve productivity, carbon performance and reduce waste, such as the use of digital design and offsite manufacturing technologies to create a larger range of building types.
Research and innovation to optimise the design and specification of buildings to reduce the materials needed in construction or repurposing, and/or to look at innovative ways of reusing waste material into new configurations (by recycling cement, steel and other carbon-intensive materials into new building material).
Research into embedding a focus on measuring and mitigating embodied and operational carbon emissions into the procurement and management of public sector infrastructure and construction projects.
Figure 19: Heat and buildings innovation needs timelines
Develop an enabling environment for system-wide decarbonisation of building stock:
- Leverage data to research and establish the real energy performance of buildings including research on factors increasing the risk of gaps between models and actuals to inform decisions and drive improvements - short term (2020 to 2025)
- Integrate retrofit solutions to deliver optimal mix of building fabric interventions and heating, cooling and storage tech for different buildings and end-users - short term (2020 to 2025)
- Develop innovative business models (such as energy as a service) and market arrangements (such as time-of-use tariffs) and improve workforce upskilling processes - short to medium term (2020 to 2030)
- Further innovation to encourage standardisation of components, contracts and installation practices - short term (2020 to 2025)
- Support organisations to develop and trial green finance options - short term (2020 to 2025)
Removing barriers to widespread energy efficiency retrofits in existing domestic and non-domestic buildings:
- Reduce upfront cost through systems engineering, including testing Whole House Retrofit and capturing real world performance to support new services - short to medium term (2020 to 2030)
- Reduce space requirements of retrofit and other energy efficiency solutions - short term (2020 to 2025)
- Develop energy reduction and efficiency solutions for hard-to-treat properties - medium term (2025 to 2030)
- Develop the supply chain models needed for deploying energy efficiency solutions at scale - short to medium term (2020 to 2030)
- Develop low-cost and transparent ways of measuring the real-world performance of energy efficiency measures (for example, though Smart Metering Enabled Thermal Efficiency Ratings) to incentivise improved performance and support new end-user services and products - short to medium term (2020 to 2030)
Developing, demonstrating, reducing costs of and de-risking low-carbon heating and cooling technologies:
- Hydrogen: Demonstrate safety, feasibility and impacts of using hydrogen as a fuel and converting existing gas network to hydrogen, for example, emissions, wider energy system impacts - short term (2020 to 2025)
- Hydrogen: Increase readiness of and further innovation for hydrogen heating appliances, for example hydrogen-ready boilers, hybrid-hydrogen heat pumps, hobs / cookers, etc. - short term (2020 to 2025)
- Hydrogen: End user trials testing user acceptance involving practical demonstrations - short to medium term (2020 to 2030)
- Heat pumps: Reduce costs & improve efficiency, for example, industrialised manufacture and supply chain innovation - short term (2020 to 2025)
- Heat pumps: Reduce disruption from installation and use of heat pumps - short term (2020 to 2025)
- Heat pumps: Develop solutions to enable the phase out high global warming potential refrigerants, including F-gases - medium term (2025 to 2030)
- Heat pumps: Trial innovative new business models to drive uptake, including flexibility services - short term (2020 to 2025)
- Heat pumps: Improve collection of performance data, including at low temperatures - short term (2020 to 2025)
- Heat pumps: Demonstrations of innovative heat pump solutions in a range of homes and at neighbourhood / district scale - short term (2020 to 2025)
- Heat pumps: Demonstrations of mass heat pump uptake in concentrated areas - short term (2020 to 2025)
- Heat pumps: Develop solutions that optimise heat pump deployment to provide wider system benefits, including demand shifting - short term (2020 to 2025)
- Bioenergy: Understand potential role of biomass and liquid biofuels for providing heat to off-gas grid properties, potentially with hybrid heat pump systems - short term (2020 to 2025)
- Anaerobic digestion: Routes to improve biomethane yield though the use of a variety of biomass feedstocks / possibility of heating through fuels from gasification - short term (2020 to 2025)
- Anaerobic digestion: address the challenge of disposing of digestate and processes to remove contaminants and finding new markets for digestate - short term (2020 to 2025)
- Anaerobic digestion: Identify mitigation technologies and techniques to address potential negative impacts of digestate, including emissions of ammonia - short term (2020 to 2025)
- Gasification: Research and innovation into feedstocks and the gasification process, with heating in buildings a possible end-use for fuels from gasification - short term (2020 to 2025)
Maximising the potential of heat networks:
- Research and trials to incentivise connections to heat and cooling networks - short term (2020 to 2025)
- Research and trials into disaggregated models of delivery or alternative business models to increase pace of delivery - short term (2020 to 2025)
- Research and trials of innovative solutions to access of heat from, for example, mine waste, geothermal, nuclear, industrial clusters and Energy from Waste installations - short to medium term (2020 to 2030)
- Research and trials to identify optimal ways of transitioning high temperature combined heat and power systems to future net-zero option - medium to longer term (2020 to 2030s and beyond)
- Research and trials to understand the role of solar thermal in heat networks - short term (2020 to 2025)
- Research and trials to understand the value of thermal stores for the wider system - medium term (2025 to 2030)
- Research to identify and develop innovative delivery models that enable effective expansion of networks in heat network zones over time - medium to longer term (2020 to 2030s and beyond)
Integrating smart, low carbon technologies and solutions:
- Develop and demonstrate standardised, cyber secure, interoperable smart systems to integrate low carbon technologies and energy efficiency at the building level - short term (2020 to 2025)
- Innovation to enable technologies in buildings to provide appropriate data to networks, system operators, suppliers and service providers - short term (2020 to 2025)
- Improve understanding, modelling and calculation methodologies for energy storage, for example, how insulation, hot water tanks and batteries can be combined to provide an optimal solution - short to medium term (2020 to 2030)
Understanding end-user behaviour to drive uptake:
- Improve understanding of deployment barriers for energy efficiency improvements, smart tech and low carbon heating for domestic and non-domestic end-users - short to medium term (2020 to 2030)
- Develop understanding of necessary support to tackle low levels of awareness, empower end-users and support uptake of low-carbon heating and energy efficiency measures - short to medium term (2020 to 2030)
- Develop understanding of any impacts of installing low carbon heating and energy efficiency measures on end-user usage habits - short to medium term (2020 to 2030)
- Further research into end user green choices, acceptance and preferences to support near-term deployment ambitions - short to medium term (2020 to 2030)
Driving down emissions associated with construction:
- Development of new construction technologies and techniques to improve design and specification, productivity, carbon performance and reduce waste - short to medium term (2020 to 2030)
- Research and innovation to optimise the design and specification of buildings to reduce the materials needed in construction or repurposing, and/or to look at innovative ways of reusing waste material into new configurations - short to medium term (2020 to 2030)
- Research into embedding a focus on measuring and mitigating embodied and operational carbon emissions into the procurement and management of public sector infrastructure and construction projects - short to medium term (2020 to 2030)
Policy ambitions:
- Decide on the long term future of heat - by middle of this decade
- Install 600k heat pumps per year - by 2028
- Potential end to high-carbon heat sources off the gas grid - 2030s
- Hydrogen neighbourhood trial, hydrogen village trial, hydrogen town - by 2023, 2025 & 2030
- As many homes as possible to meet EPC band C - by 2035
- Future homes standard - 2025
4.5 Transport
Context
Transport is the largest emitting sector in the UK. Surface transport, aviation and shipping combined accounted for 32% of the UK’s GHG emissions in 2019[footnote 39] and over recent decades efficiency gains have been offset by growth in travel demand. To meet the UK’s net zero target, the Net Zero Strategy estimates that transport emissions could need to drop by 76-86% compared to 2019, down to 23-40 MtCO2e by 2050. This will require substantial change across all transport modes supported by cross-cutting technology development to facilitate a shift in user green choices and attitudes.
The Transport Decarbonisation Plan sets out the greenprint for decarbonising the UK’s transport system. It considers how people and goods move and sets out what will be needed to deliver the required emissions reduction on the way to net zero, including through place-based solutions, technology deployments and shifts to lower carbon and active modes of transport like walking and cycling, considering the needs of the whole journey. In addition, the government is consulting on our Jet Zero Strategy, which will set out the approach and principles to deliver the ambition of decarbonising aviation. Close working with other sectors including energy infrastructure, buildings, and land use will be required as well as multiple routes to decarbonisation to mitigate risk in any one technology. The speed of the transition and complex interdependencies involved will require systems-level understanding and solutions.
Research and innovation is needed both to demonstrate and accelerate the deployment of technologies that are already close to market, and to develop and test emerging solutions that have potential to be the foundation for future advances. This is crucial in the harder to decarbonise maritime and aviation sectors, but also essential to ensure the UK retains its technological leadership in the automotive sector, one of the places where we are already seeing an acceleration in activity. It also means social research and behavioural science to accelerate the adoption of active travel and public transport and to enable the shift to zero emission vehicles as well as a better understanding of how we can provide people with better choices around travel.
Co-benefits of improved air quality and reducing adverse health impacts are likely to provide an additional incentive for change. Necessary new skills will need to be developed and widely available in time across manufacturing, supply chain and service industries. Innovation in the electricity market, infrastructure, and flexible services will be required to support decarbonisation in transport. Whilst the electrification of most surface transport will create new demand for electricity, it also provides opportunities for enhanced system flexibility through vehicle to grid technologies (see chapter 4.1.1).
Transport-related research and innovation should include the following.
- Address the transport modes, nodes and use cases where there are currently no clear or agreed solutions (the harder to decarbonise areas) alongside efforts to increase efficient and sustainable supply of resources for technologies that are close to market (such as newer battery technologies).
- Understand how we can make lives better by changing the journeys people need to make. This includes technologies that alter the need to travel, change travel modes or shift travel times, social trends which impact on commuting and work and changes to how we transport goods.
- Understand when, where and how much decarbonised energy is required for each transport mode, and for the system as a whole, now and into the future.
- Develop transport-specific energy supply technologies and other supporting infrastructure, including manufacture, as part of a place-based, decentralised wider energy system.
- Support new product development leading to manufacturing scale-up and investment, anchoring the value chain in the UK to maximise UK content and economic benefit alongside meeting net zero targets and exploring co-benefits.
- Deliver systems-level decarbonisation and modal shift, by bringing behavioural and technological solutions together at scale to meet user needs for particular geographical areas, sectors, and services.
Innovation needs include options for road freight, maritime and aviation to identify and develop best fit technology pathways for the UK by the mid-2020s; preparation of demonstration programmes and trial infrastructure which will be ready to test and understand the implementation of these new solutions in the mid-2020s to enable roll-out through the 2030s – especially where vehicles have long operational lives like trains and ships; and identifying complementary options for decreasing road emissions to meet Carbon Budget 6. This includes behaviour change, modal shift and data-driven approaches.
The medium-term (2025 to 2030) focus will be completing demonstration programmes in trucks, maritime and aviation; continuing to support the penetration of electrification throughout the light road vehicle fleet; supporting decisions on UK-wide infrastructure needs (charging infrastructure, hydrogen, ports and airports) and how they are best integrated into the wider energy system; as well as data and asset sharing in the freight and logistics system to enable better carbon efficiency in the short-term and efficient deployment of zero-emissions vehicles and other assets in the medium / longer term.
The challenges of transportation are heightened by the international context. Most modes have interfaces with other countries or at the very least similarities which have implications for standardisation of technologies, the harmonisation of regulations, and the sharing of learning and effort. For example, as a core member of the Zero-Emission Shipping Mission[footnote 40], the UK is working across the entire maritime value-chain— ships, fuel production, fuel infrastructure— through a focus on ‘green shipping corridors’.
Investments in research and innovation will also provide market opportunities for UK companies, especially in the hardest-to-decarbonise transport sectors such as shipping, aviation and heavy goods vehicles, where all countries will be facing the same challenges, and in relation to the development and commercialisation of hydrogen and sustainable aviation fuels. In addition, by pursuing an integrated approach that combines active travel and micro-mobility with behavioural science and new low emissions infrastructure, the UK can showcase systems-levels solutions and export related technologies and services to other countries.
Figure 20: System interlinkages between Transport and other sectors
Description of figure 20
Figure representing the system interlinkages between Transport and the 6 other sectors:
Natural resources, Waste and F-gases -
- Production and use of sustainable biofuels
- Low-carbon transport of agricultural goods and forestry products
Industry -
- Co-located hydrogen hubs
- Manufacture of low carbon vehicles and components
- Transport of goods and services
- Low carbon materials
Heat and buildings -
- Electric vehicle charging
- Smart home technologies
- Demand side response for grid balancing
- Low carbon transport of materials
Hydrogen -
- Hydrogen as fuel for surface transport, aviation and shipping
- Co-located hydrogen production and transport refuelling hubs
Power -
- Power demands for electric vehicles
- Vehicle-to-grid technologies
- Low-carbon transport of goods
CCUS & GGRs -
- Fuel production via biomass gasification
- Transport of CO2 to storage sites
- Offsetting of residual transport emissions
4.5.1 Transport and mobility as a system
Research and innovation: challenges and needs
Much of the innovation and deployment needed to decarbonise transport will have impacts at the system level, cutting across specific transport modes and energy vectors.
Key areas for attention include developing a better understanding of system level interdependencies and ensuring that people and place are both central to a net zero transition. Behaviour change and choice need to interact with technology innovations if we are to successfully deliver the required decarbonisation. Research into behaviour and the application of behavioural theory can build an understanding of current and future users’ needs and likely green choices and how best to influence them, informing structural changes in policies, regulation, and infrastructure. Embedding these insights into transport decarbonisation decisions will allow for more effective and targeted investment in transport research and innovation.
Enabling an integrated, multi-modal transport system
Understand how to integrate transport into local and national decarbonised energy system planning, including both the electricity system and a future hydrogen economy without impacting the government’s statutory targets to improve the environment, such as for air quality.
System analysis to identify interactions with wider systems and infrastructure, including optimal locational configurations for refuelling and charging infrastructure for different modes of transport.
Investigate the potential for co-location of assets and infrastructure to improve efficiencies.
Research into the role of different policy and regulatory frameworks, such as carbon pricing and emissions zones, to impact choices and acceptance of actors across the supply chain and end-users.
Research into skills which support the transition in transport across the board, some of which are not visible yet.
Research into new approaches for sharing and integrating data to enable a smoother transition (such as in freight) and enable holistic understanding of the role of different interventions on overall carbon impact.
Understanding how to unlock efficiency benefits in freight and logistics by better leveraging of data and sharing.
Accelerating the adoption of active travel and public / shared transport
Innovations in the design of buildings, the wider built environment, including towns and cities, that support more sustainable green choices and enable low carbon forms of travel.
Research to encourage the safe shift to more active forms of travel such as cycling, walking and newer forms of micro-mobility, considering how user needs and experiences are shaped and any barriers to making that shift. Also understanding co-benefits such as improved cardiovascular health and improved air quality and how these can provide additional incentives for people to embrace behavioural shifts.
Understanding the potential impacts of innovations which impact on transport use, such as digital technologies.
Addressing regional needs and place-based approaches
Ensure technological and market changes are built around an understanding of end-users and the communities in which they live and work.
Understand local / regional needs and opportunities so that decarbonisation approaches build on existing skills to support local economic growth.
Understand transport’s role in improving equity across the UK, including equitable solutions for rural areas and maximising potential for new skill development.
Understand how decarbonisation interventions can deliver wider co-benefits in terms of air quality, noise and health, including for vulnerable groups.
Understand the benefits of the colocation of transport energy infrastructure, such as marine and aviation refuelling which could share raw materials or other aspects of operations.
Improving efficiency and reducing carbon intensity / eliminating emissions for production of vehicles, vessels and infrastructure
Fuel and energy efficiency improvements, new materials and light weighting improvements selected on the basis of whole life cycle analysis.
Embedding circular economy approaches throughout product lifecycle, including innovations in design, retrofit, and reuse.
Application of low carbon construction techniques, skills and materials for new transport infrastructure – concrete, steel, road surfaces, etc.
Improve data usage and connected travel that actively reduces journey length and improves journey efficiency across modalities.
Understanding and enabling hydrogen’s role in transport
(Hydrogen production is covered in section 4.2.2).
Understand the role of transport in the hydrogen economy and vice versa, including the role of a centralised versus decentralised hydrogen production system.
Understand societal barriers to uptake and use of hydrogen vehicles.
Identifying requirements for fuel handling, distribution and refuelling technologies for hydrogen across all modes and for ammonia within the maritime sector and (potentially) aviation.
Accelerate hydrogen research, development and demonstration in core enabling technologies, such as fuel cells.
Understanding and demonstrating the value of hydrogen storage onboard all vehicles, including secondary engine solutions.
Hydrogen safety and security research, standards, and technologies.
Potential hydrogen role in synthetic fuel production.
Demonstration of end-use at scale, including hydrogen refuelling and use in multi-modal operational trials.
Supporting the urgent roll-out of electrification across all transport modes
Understanding and designing electricity storage, distribution, and recharging technologies across all modes and impact of large-scale electrification of transport on the power system.
Developing battery technologies for heavier modes with higher power requirements.
Developing more sustainable battery technologies which are less reliant on scarce mineral supply chains, and innovation to mitigate end-of-life impacts (including recycling and repurposing of batteries).
Development of new electric power trains and drives and other key components and subsystems of electromobility.
Large scale trials and demonstration of successful system level integration to de-risk adoption of technology for new users.
Understanding the medium and long-term behavioural changes of travellers as a result of COVID-19
Working to lock-in beneficial changes in travel behaviour, for example, increases in cycling, and countering less beneficial changes like increased car usage.
Undertaking long term behavioural studies to understand the impact of COVID-19 on people’s travel patterns, including a move to more flexible working resulting in less demand for commuting, or any aversion of crowded spaces which could result in reduced use of public transport and how this will impact upon travel planning and future policy development.
4.5.2 Land transport
Research and innovation: challenges and needs
Surface transport accounted for 22% of total UK GHG emissions in 2019[footnote 41]. Electrification is expected to be the principal decarbonisation solution, but other low carbon fuels, particularly hydrogen, may have an important role to play particularly for HGVs, other larger vehicles, and for trains on hard-to-electrify lines. Research and innovation challenges focus on technologies for different transport modes, how to design and build new refuelling and recharging infrastructure and how to integrate electric vehicles with the wider electricity system. Complementary approaches to reducing emissions from roads will also be key for meeting Carbon Budget 6.
Supporting the development and deployment of zero emission road vehicles
For light road vehicles:
- Improving the efficiency and scaling of Electric Vehicle (EV) manufacture, including relevant technologies for the EV supply chain.
- Advances in zero emission on-vehicle technologies to improve EV range, efficiency and capability.
- Trialling of EV infrastructure improvements including charging and vehicle to grid (V2G) integration, also flexible / smart charging.
- Accelerating advances in lithium-ion battery technology, such as lifespan, range, recyclability and weight.
- Development of novel battery technologies which can improve on the environmental impacts or other aspects of performance of current technologies later in the transition to net zero.
- Make smart charging attractive and accessible to diverse users, through trial products and incentives, as well as developing cyber secure and interoperable smart charging products.
- Improve the user experience of zero emission vehicles through advances in range capability, accessibility, cost reduction and other aspects of usability.
For buses and coaches:
- Research to understand how to deploy hydrogen and electric buses to respond to local factors such as climate, topography, population density and travel needs.
- Research in decarbonised coach solutions to meet coach-specific requirements.
For HGVs, municipal vehicles and non-road machinery:
- Testing and demonstration of candidate technologies including hydrogen, battery and electric road systems (such as overhead catenary lines) to support the deployment of infrastructure at scale and reduce other environmental impacts.
- Decarbonised agricultural, construction, and mining vehicles and supporting refuelling / recharging solutions.
- Fuel cell flexibility, efficiency, reliability and materials sustainability.
- Mega-charging for HGVs.
Complementary approaches to roads decarbonisation
Research into approaches to drive modal shift.
Research into changes in travel behaviour.
Data-driven techniques to reduce road transport emissions.
Decarbonising railways
Develop and demonstrate solutions for lines which are uneconomical to electrify and where rolling stock needs to run across the whole network, as in freight and maintenance.
Demonstration and evaluation of efficient hydrogen trains and cost-effective hydrogen rail infrastructure.
Investigate hydrogen distribution by rail to support other modes and sources of demand, including hydrogen rail depots and their role as potential hydrogen economy nodes.
Trial and demonstrate zero emissions rail freight infrastructure, including warehouses and depots.
4.5.3 Aviation and maritime
Research and innovation: challenges and needs
Aviation emissions (including the UK’s share of international aviation) have more than doubled since 1990[footnote 42]. Maritime emissions are growing as a proportion of all transport emissions, with domestic shipping producing more GHGs in 2019 than buses and rail combined[footnote 43]. If UK international shipping emissions are also included, the maritime sector produces almost 3 times the GHG emitted in 2019 by buses and rail combined.
Both aviation and maritime are amongst the most difficult-to-decarbonise sectors of the economy with most low carbon and zero-carbon solutions at very early stages of development. As a result, research and innovation is particularly crucial in these sectors and a variety of potential solutions need to be developed and tested over the coming decade, to enable the best options to be scaled up. This includes Sustainable Aviation Fuels obtained from low carbon feedstocks which could be combined with existing conventional jet fuel to reduce emissions. The transnational nature of aviation and maritime underscores the importance of global collaboration to develop fully interoperable solutions for the refuel and recharge of aircraft and vessels.
Developing net zero emission flight and associated operations
Integrated research and innovation activity across aerospace sector capabilities including, improving efficiencies, zero emissions flight, Sustainable Aviation Fuels markets and removals and influencing end-user choices.
Zero emissions aircraft and airside vehicles across vehicle type, load and journey length, with care to avoid increases in non-CO2 greenhouse gases.
Preparing UK airports for adoption of net zero emission aircraft and zero CO2 airside operations (ground vehicles and refuelling infrastructure).
Facilitate continued UK research and innovation on aerospace manufacturing.
Zero emissions flight airfields and operations:
- Zero emission flight airport infrastructure research and innovation.
- Continued development of more efficient and zero emission aircraft.
- Certification and infrastructure requirements for large zero emission commercial passenger aircraft.
- Continued modernisation of UK airspace.
Develop and trial sustainable aviation fuel production in the UK. E-fuels, gasification and waste lipids present options, but each has wider system implications which need to be understood.
Decarbonising maritime
Deliver research and innovation to unlock industry investment in clean maritime technologies, tackling supply and demand side barriers as well as developing infrastructure and user confidence. This includes:
- Trials and demonstrations to accelerate the market penetration of zero emission vessels and systems, including battery electric vessels, charge points and other port infrastructure for the bunkering and refuelling of alternative fuels.
- Technology development and demonstrations of pre-commercial vessels and infrastructure using hydrogen, ammonia and other zero emission solutions without impacting on the government’s timescales to meet other statutory targets (such as air quality), enabling solutions to reach market level and building market confidence.
Demonstration of hydrogen and ammonia bunkering and dispensing at ports and at sea.
Other priorities include:
- Research and innovation for automation and other technologies which can boost energy efficiency of port related activities.
- Work to understand the energy usage at ports, including impact on the grid.
Figure 21: Transport Innovation Needs Timelines
Transport and mobility as a system
Enabling an integrated, multi-modal transport system:
- Understand how to integrate transport into local and national decarbonised energy system planning - short to medium term (2020 to 2030)
- System analysis to identify interactions with wider systems and infrastructure, including optimal locational configurations for refuelling/charging infrastructure - short to medium term (2020 to 2030)
- Investigate the potential for co-location of assets and infrastructure to improve efficiencies - short to medium term (2020 to 2030)
- Research into the role of different policy and regulatory frameworks, such as carbon pricing and emissions zones, to impact behaviour of actors across the supply chain and end-users - short to medium term (2020 to 2030)
- Research into skills which support the transition in transport across the board, some of which are not visible yet - short to medium term (2020 to 2030)
- Research into new approaches for sharing and integrating more data to enable a smoother transition, such as in freight - short term (2020 to 2025)
- Understand how to unlock efficiency benefits in freight and logistics by better leveraging of data and sharing - short term (2020 to 2025)
Accelerating the adoption of active travel and public / shared transport:
- Innovations in the design of buildings and the wider built environment that support more sustainable green choices and enable low carbon forms of travel- short to longer term (2020 to 2030s and beyond)
- Research to encourage the safe shift to more active forms of travel such as cycling, walking and newer forms of micro-mobility - short to longer term (2020 to 2030s and beyond)
- Understand the potential impacts of innovations which impact on transport use, such as digital technologies - short to longer term (2020 to 2030s and beyond)
Addressing regional needs and place-based approaches:
- Ensure technological and market changes are built around an understanding of end-users and the communities in which they live and work - short to medium term (2020 to 2030)
- Understand local / regional needs and opportunities so that decarbonisation approaches build on existing skills to support local economic growth - short to medium term (2020 to 2030)
- Understand transport’s role in improving equity across the UK, including for rural areas and maximising potential for new skill development - short to medium term (2020 to 2030)
- Understand how decarbonisation interventions can deliver wider co-benefits in terms of air quality, noise and health, including for vulnerable groups - short to medium term (2020 to 2030)
- Understand the benefits of the colocation of transport energy infrastructure, such as marine and aviation refuelling - short to medium term (2020 to 2030)
Improving efficiency and reducing carbon intensity / eliminating emissions for production of vehicles, vessels and infrastructure:
- Fuel and energy efficiency improvements, new materials and light weighting improvements selected on the basis of whole life cycle analysis - short to longer term (2020 to 2030s and beyond)
- Embed circular economy approaches throughout product lifecycle, including innovations in design, retrofit, and reuse - short to longer term (2020 to 2030s and beyond)
- Application of low carbon construction techniques, skills and materials for new transport infrastructure – concrete, steel, road surfaces, etc. - short to longer term (2020 to 2030s and beyond)
- Improve data usage and connected travel that actively reduces journey length and improves journey efficiency across modalities - short to medium term (2020 to 2030)
Understanding and enabling hydrogen’s role in transport:
- Understand the role of transport in the hydrogen economy and vice versa, including centralised vs. decentralised hydrogen production system - short term (2020 to 2025)
- Understand societal barriers to uptake and use of hydrogen vehicles - short term (2020 to 2025)
- Identify requirements for fuel handling, distribution and refuelling techs across all modes and for ammonia in maritime and (potentially) aviation - short term (2020 to 2025)
- Accelerate hydrogen research, development and demonstration in core enabling technologies, such as fuel cells - short term (2020 to 2025)
- Understand and demonstrate the value of hydrogen storage onboard all vehicles, including secondary engine solutions - short to longer term (2020 to 2030s and beyond)
- Hydrogen safety and security research, standards, and technologies - short term (2020 to 2025)
- Potential hydrogen role in synthetic fuel production - short term (2020 to 2025)
- Demonstration of end-use at scale, including hydrogen refuelling and use in multi-modal operational trials - short to medium term (2020 to 2030)
Supporting the urgent roll-out of electrification across all transport modes:
- Understand and design electricity storage, distribution, and recharging technologies across all modes and impact of electrification of transport on the power system - short to medium term (2020 to 2030)
- Develop battery technologies for heavier modes with higher power requirements - short to medium term (2020 to 2030)
- Develop more sustainable battery technologies which are less reliant on scarce mineral supply chains, and innovation to mitigate end-of-life impacts - short to medium term (2020 to 2030)
- Development of new electric power trains and drives and other key components and subsystems of electromobility - short to medium term (2020 to 2030)
- Large scale trials and demonstration of successful system level integration to de-risk adoption of technology for new users - medium term (2025 to 2030)
Behavioural changes of travellers as a result of COVID-19:
- Work to lock-in beneficial changes in travel behaviour during the pandemic, for example, increases in cycling and countering less beneficial changes like increased car usage - short term (2020 to 2025)
- Undertake long term behavioural studies to understand the impact of COVID-19 on people’s travel pattern - short term (2020 to 2025)
Policy ambitions:
- End sale of new petrol and diesel cars and vans. All new cars and vans must be zero emission at the tailpipe - 2035
- End sale of new non-zero emission HGVs (subject to consultation) - 2040
- Net zero domestic aviation (subject to consultation) - 2040
- Ambition to remove all diesel-only trains from the network - 2040
Land transport
Supporting the development and deployment of zero emission road vehicles:
- Light road vehicles: Research and innovation to improve the efficiency and scaling of EV manufacture including relevant technologies for the EV supply chain - short to medium term (2020 to 2030)
- Light road vehicles: Advances in zero emission on-vehicle technologies to improve EV range, efficiency or capability - short to medium term (2020 to 2030)
- Light road vehicles: Trial EV infrastructure improvements including charging and vehicle to grid (V2G) integration, also flexible / smart charging - short to longer term (2020 to 2030s and beyond)
- Light road vehicles: Accelerate advances in lithium-ion battery technology, such as lifespan, range, recyclability and weight - short to medium term (2020 to 2030)
- Light road vehicles: Development of novel battery technologies which can improve on the environmental or other aspects of performance of current technologies later in the transition to zero - short to longer term (2020 to 2030s and beyond)
- Light road vehicles: Make smart charging attractive and accessible to diverse users, through trial products and incentives, alongside developing cyber secure and interoperable smart charging products - short term (2020 to 2025)
- Light road vehicles: Research and innovation to improve the user experience of zero emission vehicles through advances in range capability, accessibility, cost reduction and other aspects of usability - short to longer term (2020 to 2030s and beyond)
- Buses & coaches: Research to understand how to deploy hydrogen and electric buses to respond to local factors such as climate, topography, population density - short term (2020 to 2025)
- Buses & coaches: Research in decarbonised coach solutions to meet coach-specific requirements - short term (2020 to 2025)
- HGVs, municipal and machinery: Test and demonstrate of candidate technologies including hydrogen, battery and electric road systems - short to longer term (2020 to 2030s and beyond)
- HGVs, municipal and machinery: Decarbonised agricultural, construction, and mining vehicles and supporting refuelling / recharging solutions - short to longer term (2020 to 2030s and beyond)
- HGVs, municipal and machinery: Fuel cell flexibility, efficiency, reliability and materials sustainability - short to longer term (2020 to 2030s and beyond)
- HGVs, municipal and machinery: Mega-charging for HGVS - short to medium term (2020 to 2030)
Complementary approaches to roads decarbonisation:
- Research into approaches to drive modal shift - short to medium term (2020 to 2030)
- Research into changes in travel behaviour - short to medium term (2020 to 2030)
- Data-driven techniques to reduce road transport emissions - short to medium term (2020 to 2030)
Decarbonising railways:
- Develop and demonstrate solutions for lines which are uneconomical to electrify and where rolling stock needs to run across the whole network - short to medium term (2020 to 2030)
- Demonstration and evaluation of efficient hydrogen trains and cost-effective hydrogen rail infrastructure - short to medium term (2020 to 2030)
- Investigate hydrogen distribution by rail to support other modes and sources of demand, including hydrogen rail depots and their role in a hydrogen economy - short term (2020 to 2025)
- Trial and demonstrate zero emissions rail freight infrastructure, including warehouses and depots - short to medium term (2020 to 2030)
Aviation and maritime
Developing net zero emission flight and associated operations:
- Integrated research and innovation activity across aerospace sector capabilities, including improving efficiencies, zero emissions flight, sustainable aviation fuels, markets and removals, and influencing end-user choices - short to medium term (2020 to 2030)
- Zero emissions aircraft and airside vehicles across vehicle type, load and journey length, with care to avoid increases in non-CO2 Greenhouse gases - short to medium term (2020 to 2030)
- Prepare UK airports for adoption of net zero emission aircraft and zero CO2 airside operations (ground vehicles and refuelling infrastructure) - short to medium term (2020 to 2030)
- Facilitating continued UK research and innovation on aerospace manufacturing - short to medium term (2020 to 2030)
- Zero Emission Flight airport infrastructure research and innovation early in the transition, to support other elements of a net zero aviation system - short term (2020 to 2025)
- Continue development of more efficient and zero emission aircraft - short to medium term (2020 to 2030)
- Certification and infrastructure requirements for large zero emission commercial passenger aircraft - short to medium term (2020 to 2030)
- Continue modernisation of UK airspace - short to longer term (2020 to 2030s and beyond)
- Develop and trial sustainable aviation fuel production in the UK. E-fuels, gasification and waste lipids present options, but each has wider system implications which need to be understood - short to longer term (2020 to 2030s and beyond)
Decarbonising Maritime sectors:
- Deliver research and innovation activity to tackle supply and demand side barriers as well as developing infrastructure and user confidence - short to medium term (2020 to 2030)
- Trials and demonstrations to accelerate the market penetration of zero emission vessels and infrastructure such as battery vessels, chargepoints and cold ironing - short to medium term (2020 to 2030)
- Technology development and demonstrations to enable pre-commercial solutions including hydrogen and ammonia fuel cell systems to reach market level - short to medium term (2020 to 2030)
- Demonstration of hydrogen and ammonia bunkering and dispensing at ports and at sea - short to medium term (2020 to 2030)
- Research and innovation for automation and other technologies which can boost energy efficiency of port related activities - short to medium term (2020 to 2030)
- Work to understand the energy usage at ports and provision of cold ironing (provision of shoreside power for ships at berth) - short term (2020 to 2025)
4.6 Natural resources, Waste and F-gases
Context
Achieving net zero will require the strategic and sustainable management of land, waste and natural resources - including agriculture, biomass production, treescape and forestry, soil and peatland management, the marine and coastal environment, waste and recycling, urban developments and infrastructure, wastewater treatment and fluorinated gases (F-gases).
These sectors will need to contribute to net zero in 4 ways.
- By reducing direct emissions. Currently around 10% of the UK’s total GHG emissions come from agriculture and around 4% from the waste sector[footnote 44]. These are mostly non-CO2 emissions such as methane from livestock and decomposing waste, and nitrous oxide from the application of fertiliser and organic materials to land.
- By sequestering carbon dioxide from the atmosphere and helping to offset residual emissions from other hard-to-abate sectors. Afforestation and changes in land management offer routes to achieve this objective, but air quality currently has a significant impact on tree health, which may impact on their ability to sequester carbon at the rate expected.
- By providing sustainable biomass for energy generation directly and in the production of other fuels (such as sustainable aviation fuels) and the wider bioeconomy (such as wood in construction and for bio-based plastics). This can reduce emissions from materials with high GHG emissions, such as cement, and provide additional storage of carbon, although the risks of wider negative impacts from unsustainable biomass use must be carefully managed.
- By reducing the use of F-gases in equipment such as refrigeration and air-conditioning (driven by a switch to alternative gases and technologies).
Delivery of these aims must be achieved at the same time as improving biodiversity, air quality, water quality, natural capital, ecosystem services and resilience to climate change. The 25 Year Environment Plan sets out the UK’s goals for improving the environment within a generation. It aims to deliver cleaner air and water, protect species and habitats, tackle waste and soil degradation and help to mitigate and adapt to climate change. The plan, which incorporates the government’s statutory targets, aims to deliver once-in-a-lifetime changes to reform agriculture and manage land more sustainably.
Ongoing research and monitoring will be needed to provide the evidence base to guide policy and related decision making over time. This will require an integrated, systems-based approach that takes account of emissions reduction alongside the need to meet these other statutory environmental targets and social and economic goals. There will be co-benefits and trade-offs between different objectives, and these need to be managed responsibly with a broad understanding of wider impacts across different sectors and places. Social and behavioural considerations and a focus on environmental science will be as important as technology in driving innovation and the changes needed for net zero, such as changes in consumer demand for food, public acceptability of new products, and the willingness of farmers and land managers to adopt new approaches. As a result, research and innovation must focus on people as much as technology and environmental science.
Scientific breakthroughs in nutrition, genetics, informatics and big data, remote sensing, engineering, robotics, meteorology, and monitoring technologies are playing a key part in developing technologies to transform traditional practices. Business opportunities exist in the UK agri-tech sector, which serves a growing international market; in the biosciences and biotechnologies sector; in carbon professional services, such as selling expertise in low carbon farming practices; and in monitoring, reporting and verifying emissions. Carbon sequestration projects may also provide future opportunities when further developed.
Figure 22: System interlinkages between Natural Resources, Waste and F-gases and other sectors
Description of figure 22
Figure representing the system interlinkages between Natural resources, Waste and F-gases and the 6 other sectors:
Transport -
- Production and use of sustainable biofuels
- Low-carbon transport of agricultural goods and forestry products
Industry -
- Bioenergy used to fuel industrial processes and BECCS
- Biomass supply for industries, for example, paper, food, construction
- Circular economy & material flows
Heat and buildings -
- Bioenergy for hard- to-decarbonise buildings
- Biogas grid injection
- Biogenic building materials
Hydrogen -
- H2-BECCS (hydrogen production from biomass using CCUS)
- Water demand for hydrogen production
Power -
- Biomass supply for bioenergy, including BECCS
- Electrification of agricultural equipment
CCUS & GGRs -
- Afforestation, soil carbon, peatland restoration
- Capture of GHGs from landfill and other waste processes
4.6.1 An integrated and dynamic approach to land-use
Research and innovation: challenges and needs
There are multiple demands on land that influence how it is used and the urgency of net zero means that actions may need to be taken before all wider impacts are adequately understood. Compounding this challenge is the complexity of interactions between land management decisions, impacts on society and the natural environment and overall GHG emissions – including those outside the UK. Land-use planning is therefore a key consideration for research and innovation. A flexible and dynamic approach, that recognises the tensions and trade-offs in decision making will be essential, underpinned by the best possible understanding of the multi-objective systemic nature of land use and management.
Land use allocation & planning considerations
Develop the tools and capabilities to inform land-use decisions and policy interventions at national and local scales, taking into account the interplay between land-use, end user preferences, land manager acceptability, policy and regulatory frameworks, incentives and technologies:
- Research on green financing framework and practical applications of blended finance mechanisms.
- Research on the economic values of protected landscapes.
- Research on Monitoring and evaluation of landscapes policies.
Understanding system level greenhouse gas emissions and environmental impacts
Systems research to assess solutions within land, social, economic and environmental constraints, and considering a broad range of impacts including on natural capital and climate resilience:
- Research into agroecological and integrated farm management practices.
- Understanding the impact historical land use or pollution deposition may have on the ability to deliver carbon sequestration goals.
Develop modelling and datasets for greenhouse gas impacts of agricultural processes, forestry, peat and soils, to understand impact of changes at system level in conjunction with other environmental pressures, such as air quality.
Effecting sustainable and responsible land use change and effects on economic growth / levelling-up agenda
Research and innovation to identify the social, economic and cultural drivers of land use, barriers to sustainable land use change, and assess the options to effect these changes.
Research and innovation into markets and financing to drive tree planting, perennial energy crops and short rotation forestry for bioenergy and the wider bioeconomy, peatland restoration and other forms of sustainable land use and tracking compliance / verifying delivery of intended reductions.
Research to measure profitability of sustainable and responsible farming systems on peat and soils and how food production will be impacted.
Research to review how existing land use models are informing economic growth decisions at both national and local level:
- Understand how skills programmes and education strategy can support environmental goals and green growth.
- Research on environmental taxation.
- Research on environmental impacts of new trade agreements.
- Research on corporate natural capital accounting.
- Research on barriers to accessing nature for certain demographics.
4.6.2 Forests, peatland and the marine environment
Research and innovation: challenges and needs
The UK’s natural assets, such as its treescape, forests, soils, peatlands and marine environments are important habitats for a range of species and provide multiple benefits to society, including carbon storage and sequestration, low carbon energy generation and feedstock production, biodiversity and improved natural capital assets. Net zero will require changes to the management of these environments and research is required to better understand these changes and their impacts, and new approaches and solutions will need to be developed and demonstrated.
Trees act as a natural carbon sink and are essential to meet long term targets. The government is committed to increasing tree-planting in the UK to 30,000 hectares (ha) per year by 2025 and alongside this there is a need to improve the management of existing woodlands. This must be underpinned by research into appropriate species selection and responsible land management practices.
Peat is the UK’s largest terrestrial carbon store. Farmed lowland peat is currently responsible for 86% of England’s peatlands carbon emissions, but is also some of the most productive agricultural land. Better management of lowland and upland peatlands will see a range of co-benefits alongside climate change mitigation. However, significant areas of peatland exceed safe thresholds for nitrogen deposition and ammonia exposure which damages the mosses responsible for peat production. The government is committed to restoring 35,000 ha of peat in England by 2025.
Healthy soils underpin a range of environmental, economic and societal benefits including carbon storage and biodiversity. The 25 Year Environment Plan sets the goal of sustainably managing all of England’s soils by 2030. Developing a healthy soils indicator, soil structure and soil health measuring and monitoring schemes will enable the government to deliver on this target; research into methodologies and baseline data sets will support the development of suitable metrics and indicators.
Certain marine and coastal habitats, including saltmarsh, seagrass and marine sediments, are important long-term carbon stores. These habitats can be lost or damaged by human activities and their area is being squeezed by sea level rise, which can contribute to carbon emissions, whilst habitat creation and restoration could contribute to carbon removals whilst also providing a range of co-benefits for biodiversity and climate adaptation. Currently 38% of UK waters are in Marine Protected Areas, covering the majority of saltmarsh and seagrass habitats, with the government now taking steps to ensure these are effectively protected. The marine environment also hosts infrastructure such as offshore wind, and this development must be pursued in ways that protect or enhance ecosystems.
Sustainably expanding and managing forests and the wider treescape
Develop and trial improved ground based and remote sensing technologies and modelling for carbon storage calculations, including forest soils and trees outside woodlands. Expand long term plot monitoring to resolve uncertainties.
Understand the variability and dependencies between different woodland types and silvicultural dependencies (rewilding, natural colonisation, etc.) including to changing climatic and environmental conditions.
Understand silvicultural and arable agroforestry impacts on biomass and soil carbon, soil health and wider greenhouse gas balances.
Manage and trial strategies to minimise damage to forestry from pests and pathogens.
Field trials of short rotation forestry, including using exotic species for biomass.
Develop and trial innovative finance models for land managers to transition to forestry.
Develop and trial innovative supply chains for planting materials well adapted to the UK.
Understand social and behavioural drivers and barriers, for example, land ownership, acceptability, skills, finance, incentives, co-benefits.
Collaborative R&D with the commercial forestry and building sectors to increase supply and demand of timber in built infrastructure.
Establish robust estimates of the abatement delivered by harvested wood products in use through carbon storage and product substitution.
Developing increased resilience of forest ecosystems to climate change impacts
Improved understanding of the resilience of woodlands and trees to climate change, including impacts of pests, diseases and other environmental pressures such as air quality.
Genomic sequencing of tree populations and mapping of characteristics to improve enhancements beneficial to future circumstances.
Research into the soil bacteria characteristics of different forest types, to understand how bacterial communities enhance (or disadvantage) resilience in different ways.
Research into fungi characteristics of different forest types, to understand how fungi enhance (or disadvantage) resilience in different ways.
Restoring sustainably and managing peatlands
Research into, and the development of, sustainable lowland peat management options that allow for productive and profitable agriculture to take place while water levels are raised, to sustain healthy and wildlife rich peatlands which provide ecosystem services such as carbon sequestration and flood mitigation and which do not lead to other negative impacts.
Understanding the tensions and trade-offs between food production, greenhouse gas emission abatement and peat loss.
Improved evidence on greenhouse gas balances of peatland restoration establishing the rate of deployment, scientific uncertainty, associated costs and trade-offs.
Better understanding of optimal landscape scale water management for wetter peat (to reduce emissions) and mitigating flood risk.
Innovative research to assess alternative approaches to peatland horticulture to enable an expanded sector while decreasing land-use emissions.
Managing soils for improved soil health and resilience
Researching and developing suitable data collection protocols and baseline data sets to develop a healthy soil indicator.
Develop and pilot a soil structure measuring and monitoring method as a key physical element of the healthy soil indicator.
Research into alternative farming methods, such as regenerative agriculture and agroforestry¸ to establish the soil health and potential carbon sequestration benefits of these systems.
Research to understand the potential requirements of a Soil Carbon Code and the potential for a future carbon farming scheme in England.
Sustainably managing the marine environment
Research into greenhouse gas emissions and removals from coastal wetlands, including impacts of coastal erosion, wetland restoration, air quality, water quality, climate change, and sea-level rise. Including improved greenhouse gas accounting and reporting, with clear assessment of additionality and permanence of changes in carbon fluxes and stores.
Assess the scale and direction of change in carbon storage and sequestration to human activities (for example, trawling, dredging, coastal development) and how to accurately account for these. Understand the impact of different management interventions and ecosystem recovery on carbon fluxes and stores.
Research and long-term monitoring to understand impacts of decarbonisation infrastructure and habitat change on other users of marine spaces, for example, recreation, fishing, shipping, infrastructure development.
Innovation in design and construction of offshore infrastructure (for example, offshore wind, 5G phone sites) to minimise environmental impacts. Also research into social acceptance of offshore programmes and the financial implications of building offshore versus on land.
Research into sustainable salt and fresh-water aquaculture systems. Identifying and measuring the benefit of ecosystem-based approaches to aquaculture on ecosystem functioning and services (for example, carbon storage and sequestration, water filtration, nutrient cycling). Better understanding the solutions required to minimise and mitigate the environmental impacts (for example, disease, escapees, discharge) of existing aquaculture systems.
4.6.3 Food and biomass
Research and innovation: challenges and needs
Over 60% of agricultural emissions are produced by livestock with the rest coming from crop production and fuel use in agriculture. Solutions are needed to reduce these emissions whilst also addressing other land-based challenges such as food security. Research and innovation needs to address both the supply and demand of agricultural products, and to develop solutions that support sustainable choices and acceptance of end-users and farmers. This may include non-traditional forms of production including vertical agriculture and lab-based production of alternative proteins.
The current biomass resource supply is diverse. A significant portion of biomass for use in energy generation and the wider bioeconomy is sourced from wastes and residues from the agriculture, forestry and waste sectors[footnote 45]. While the majority of biomass used in the production of renewable electricity and heat is from domestic sources, imported wood pellets are also important feedstocks. Most feedstocks used for renewable transport fuels derive from international sources.
Increasing the UK’s domestic supply of sustainable biomass feedstocks (principally perennial energy crops and short rotation forestry) could support decarbonisation efforts through nature-based carbon sequestration. A number of research and innovation challenges need to be overcome to achieve a scale-up in domestic biomass. This also needs to be carefully managed so that using organic materials for energy does not direct resources away from agriculture and forestry or lead to unintended negative environmental impacts, including the ability to meet statutory environmental targets.
Sustainable production of food, perennial energy crops and short rotation forestry
Research into novel technological approaches to decrease agricultural emissions and enhance sustainable production to meet food demand:
- Transformational approaches to food production including (but not limited to) vertical agriculture, urban farming, synthetic alternatives to high carbon food products, agroecology, regenerative agriculture, no-till systems, etc.
- Precision farming solutions to monitor all aspects of animal and crop production including health, nutrition and performance to optimise management and reduce inputs. To include artificial intelligence, sensors, autonomous systems and robotics.
- Investments in both conventional and advanced breeding technologies in crop and plant production to build resilience, improve sustainability and enhance productivity.
- Animal breeding, nutrition and biology to minimise enteric methane as well as nitrogen excretion, ammonia and carbon dioxide; minimising emissions per kg meat.
- Technologies that decrease emissions from animal housing, such as methane capture, and from waste storage and application such as slurry storage and low emission spreading.
- Technologies and techniques which reduce the impact of ammonia and other pollutants on sensitive habitats identified as areas for carbon sequestration.
- Sustainable crop feeds, fertilisers, pesticides and herbicides (or alternatives).
- Reduce energy consumption in agricultural production by low carbon machinery, minimum till cultivations, alternative energy sources, etc.
- Improved ground based and remote sensing technologies and modelling for robust carbon storage calculations for agricultural land.
Research to improve understanding of maintenance of soil systems to deliver multiple functions required from soil and developing an improved and efficient soil microbiome.
Research into land management to achieve best use of different types of land.
Advances in measuring and monitoring of emissions and environmental impacts from the whole agri-food supply chain, as well as social and economic impacts.
Advances in plant breeding (including through biotechnology) to increase productivity, resilience to climate change, and to minimise the use of chemicals.
Demonstration of novel systems and approaches:
- Demonstrator farms or regions to understand changes at system level, for example, agroforestry, paludiculture, novel ruminant housing systems.
- Field and farm scale data collection and sensor development.
Understand economic and behavioural barriers to change and how these can be overcome to support farmers and land-managers transition to net zero.
Research and pilot green finance and new farm business models to enable adoption and innovation of sustainable farming practices.
Sustainable consumption
Development of models to rapidly reflect changes in food demand.
Develop metrics which can monitor and communicate embedded emissions in consumer goods.
Continue research to better understand the health, environmental and resilience impacts of changing end-user preferences and markets.
Research into social drivers of consumption and wastage and mechanisms to incentivise change.
Developing a sustainable bioeconomy
(See also section 4.1.4).
Research to help develop and scale-up a sustainable and reliable supply of UK produced biomass:
- Including forestry, second generation energy crops (meaning non-food, lignocellulosic crops), agricultural residues, wastes and novel feedstocks.
- Breeding to increase varieties and improve feedstock quality.
- Better greenhouse gas lifecycle assessments of the production of different types of biomass feedstock and means to reduce these impacts, such as the use of bioenergy with carbon capture and storage (BECCS).
- Understand locational / regional impacts on environmental services.
- Understand and mitigate wider sustainability impacts.
- Develop understanding of where bio-derived chemicals can displace fossil fuels in the medium-term; supporting these supply chains.
4.6.4 Waste and F-gases
Research and innovation: challenges and needs
Emissions from waste have reduced substantially since 1990 but further action is required to tackle methane from the decomposition of biodegradable waste in landfill sites, emissions produced from the treatment of wastewater, and emissions from the biological treatment, composting and incineration of waste. Innovation is required to support the reduction of waste to help meet government targets, turn unavoidable waste into useful products and to improve and deploy anaerobic digestion technologies.
F-gases account for around 3% of total UK GHG emissions[footnote 46] and hydrofluorocarbons (HFCs) – used for applications such as refrigeration and air-conditioning – are by far the biggest source. The UK has a target to cut HFCs by 79% by 2030 and has an international obligation for an 85% cut in HFC consumption by 2036, which requires the adoption of alternative gases and technologies that use them.
The UN Montreal Protocol Parties have committed to explore options to expand the global coverage of atmospheric monitoring of ozone-depleting substances and HFCs, to tackle illegal emissions of CFC-11 and safeguard the successes of the Montreal Protocol in ozone layer protection and climate change mitigation. The UK has world leading expertise on atmospheric monitoring which provides an opportunity to contribute to this work.
Reducing waste and minimising emissions
Anaerobic digestion (AD) technology:
Research ammonia emissions mitigation technology; also understand the wider impacts of digestate, including on air, water and soil quality and the impact on the government’s other statutory targets.
Research into improved and more efficient AD, as described in section 4.4.
Landfill:
In depth review of landfill waste codes and their sources to allow targeted policies for biodegradable waste streams, with particular focus on commercial waste streams and construction and demolition waste.
Improved measurement of landfill emissions to allow a move away from estimates to empirical data, allowing capture of all landfill site-specific improvements and targeted interventions to reduce emissions at worst performing sites.
Investigate relationship between woodlanding and soil oxidation of methane, to free up more land to meet tree-planting targets, while simultaneously reducing landfill emissions through bio-covers.
Biological Treatmen:
Research policy options around reducing emissions from biological waste treatment, establishing technological, behavioural and economic barriers. This could also have impacts in the agriculture and wastewater sectors of the inventory and provide better evidence for future bioenergy strategies.
Waste reduction:
Improve understanding of carbon impacts of waste prevention, resource efficiency, waste treatment and biodegradable waste policies.
Research technological, behavioural and economic barriers to reducing plastic use, and increasing recycling (‘reduce, reuse, repair, recycle’), including support for the development of bio-based and genuinely biodegradable plastics.
Research into optimising food production, quality, shelf life, improving resource use efficiency and influencing end-user choices to reduce food waste and waste associated with production, processing and across the supply chain.
Reducing process emissions and energy use in the wastewater treatment sector
Research and modelling to deliver emissions savings from the wastewater component (particularly industrial) of the UK Inventory through data improvements (both gathering of data and refining calculations).
Widescale monitoring of emissions from wastewater treatment plants to be rolled out to better understand sources coupled with optimisation of current practices to minimise fugitive greenhouse gas emissions.
Research and trials to better understand and then enable commercial deployment of novel and alternative treatment processes including anaerobic treatment, Membrane Aerated Biofilm Reactor and alternative processes for ammonia removal.
Research into the barriers and implications of upgrading the UK water sector to advanced digestion processes.
Research and options appraisal into the costs and benefits of different mitigation options available in the wastewater system, including new technologies, energy recovery possibilities (such as microbial fuel cells) and changes to data gathering (such as on private wastewater emissions).
Minimising UK F-gas emissions
Research into the technical feasibility and cost-effectiveness of low-Global Warming Potential F-gases and alternatives to F-gases, with consideration of their energy efficiency and domestic and international standards and building and safety rules relevant to their uptake.
Enhancing the global coverage of atmospheric monitoring of Montreal Protocol controlled substances
Research to improve the global coverage of atmospheric monitoring of ozone depleting substances and hydrochlorofluorocarbons by:
- Identify the gaps in global coverage of atmospheric monitoring and suitable locations for monitoring sites
- Pilot collaborative efforts to enhance atmospheric monitoring of controlled substances, including flask sampling or high frequency monitoring stations
Figure 23: Natural resources, waste and F-gases innovation needs timelines
An integrated and dynamic approach to land-use
Land use allocation & planning considerations:
- Develop the tools and capabilities to inform land-use decisions and policy interventions at national and local scales, including research on green financing, economic values of protected landscapes and monitoring and evaluation of landscape policies - short term (2020 to 2025)
Understanding system level GHG emissions and environmental impacts:
- Systems research to assess solutions within land, social, economic and environmental constraints - short to medium term (2020 to 2030)
- Research into agroecological and integrated farm management practices and impacts on carbon sequestration goals - short to medium term (2020 to 2030)
- Develop modelling and datasets for GHG impacts of agricultural processes, forestry, peat and soils, to understand impact of changes at system level - short to medium term (2020 to 2030)
Effecting sustainable and responsible land use change and effects on economic growth / levelling-up agenda:
- R&I to identify social, economic and cultural drivers of land use, barriers to sustainable land use change, and assess the options to effect these changes - short term (2020 to 2025)
- R&I into markets and financing to drive tree planting, biomass cropping, peatland restoration and other forms of sustainable land use and track compliance - short term (2020 to 2025)
- Research to measure profitability of sustainable farming systems on peat and soils and how production will be impacted - short term (2020 to 2025)
- Research to review how existing land use models are informing economic growth decisions at both national and local levels - short term (2020 to 2025)
Policy ambitions:
- Eliminate food waste from land-fill in England - by 2030
- Eliminate avoidable waste - by 2050
- 30k hectares trees planted each year - by 2025 (and potentially beyond)
- Delivery of the Environmental Land Management Scheme - by 2025
- Delivery of the Biomass Strategy (BEIS) - by 2023
- Nature for Climate Fund set a target for 35k hectares of peat restoration in England - by 2025
- 85% cut in hydrofluorocarbon consumption - by 2036
Forests, soil, peatland and the marine environment
Sustainably expanding and managing forests and the wider treescape:
- Develop and trial improved ground based and remote sensing technologies and modelling for carbon storage calculations - short term (2020 to 2025)
- Understand the variability and dependencies between different woodland types and silvicultural dependencies (rewilding, natural colonisation, etc.) - short term (2020 to 2025)
- Understand silvicultural and arable agroforestry impacts on biomass and soil carbon, soil health and wider Greenhouse Gas balances - short term (2020 to 2025)
- Manage and trial strategies to minimise damage to forestry from pests and pathogens - short to longer term (2020 to 2030s and beyond)
- Field trials of short rotation forestry, including using exotic species for biomass - short to medium term (2020 to 2030)
- Develop and trial innovative finance models for land managers to transition to forestry - medium term (2025 to 2030)
- Develop and trial innovative supply chains for planting materials well adapted to the UK - short to medium term (2020 to 2030)
- Understand social and behavioural drivers and barriers, for example, land ownership, acceptability, skills, finance, incentives, co-benefits - short term (2020 to 2025)
- Collaborative R&D with the commercial forestry and building sectors to increase supply and demand of timber in built infrastructure - short to medium term (2020 to 2030)
- Establish robust estimates of the abatement delivered by harvested wood products in use through carbon storage and product substitution - short term (2020 to 2025)
Developing increased resilience of forest ecosystems to climate change impacts:
- Improved understanding of the resilience of woodlands and trees to climate change, including impacts of pests, diseases and environmental conditions - short to medium term (2020 to 2030)
- Genomic sequencing of tree populations and mapping of characteristics to improve enhancements beneficial to future circumstances - short to medium term (2020 to 2030)
- Research into the soil bacteria characteristics of different forest types and how bacterial communities enhance (or disadvantage) resilience - short to medium term (2020 to 2030)
- Research into the fungi characteristics of different forest types and how fungi enhance (or disadvantage) resilience - short to medium term (2020 to 2030)
Restoring sustainably and managing peatlands:
- Research and development of lowland peat management within a productive farming system to reduce emissions, enhance agricultural production and understand trade-offs between food production, wildlife, ecosystem services, Greenhouse Gas emission and peat loss - short to medium term (2020 to 2030)
- Improved evidence on Greenhouse Gas balances of peatland restoration establishing the rate of deployment, scientific uncertainty, associated costs and trade-offs - short to medium term (2020 to 2030)
- Better understanding of optimal landscape scale water management for wetter peat (to reduce emissions) and mitigating flood risk - short to medium term (2020 to 2030)
- Innovative research to assess alternative approaches to peatland horticulture to enable an expanded sector while decreasing land-use emissions - short to medium term (2020 to 2030)
Managing soils for improved soil health and resiliance:
- Research and develop suitable data collection protocols and baseline data sets to develop a healthy soil indicator - short to medium term (2020 to 2030)
- Develop and pilot a soil structure measuring and monitoring method as a key physical element of the healthy soil indicator - short to medium term (2020 to 2030)
- Research alternative farming methods such as regenerative agriculture and agroforestry to establish soil health and potential carbon sequestration benefits - short to medium term (2020 to 2030)
- Research to understand the potential requirement of a Soil Carbon Code and the potential for a future carbon farming scheme in England - short to medium term (2020 to 2030)
Sustainably managing the marine environment:
- Research into Greenhouse Gas emissions and removals from coastal wetlands, including coastal erosion, wetland restoration; and improved Greenhouse Gas accounting and reporting - short to longer term (2020 to 2030s and beyond)
- Assess scale and direction of change in carbon storage and sequestration to human activities; understand the impact of different management interventions and ecosystem recovery on carbon fluxes and stores - short to medium term (2020 to 2030)
- Research and long-term monitoring to understand impacts of decarbonisation infrastructure and habitat change on other users of marine spaces - short to medium term (2020 to 2030)
- Innovation in design and construction of offshore infrastructure (for example, offshore wind, 5G phone sites) to minimise environmental impacts. Also research into social acceptance and financial implications of building offshore versus on land - short to medium term (2020 to 2030)
- Research into sustainable salt and fresh-water aquaculture systems, including solutions to mitigate the environmental impacts (for example, disease; escapees; and discharge) of existing aquaculture systems - short to medium term (2020 to 2030)
Food and biomass
Sustainable production of food, perennial energy crops and short rotation forestry:
- Transformational approaches to food production including (but not limited to) vertical agriculture, urban farming, synthetic alternatives, agroecology, no-till systems - short to medium term (2020 to 2030)
- Precision farming solutions to monitor all aspects of animal and crop production including health, nutrition and performance to optimise management and reduce inputs - short to medium term (2020 to 2030)
- Investments in both conventional and advanced breeding technologies in crop and plant production to build resilience, sustainability and productivity - short to medium term (2020 to 2030)
- Animal breeding, nutrition and biology to minimise enteric methane, nitrogen excretion, ammonia and carbon dioxide; optimising emissions per kg meat - short to medium term (2020 to 2030)
- Technologies that decrease emissions from animal housing, such as methane capture, and from waste storage and application - short to medium term (2020 to 2030)
- Technologies and techniques that reduce impact of ammonia and other pollutants on sensitive habitats identified as areas for carbon seqestration - short to medium term (2020 to 2030)
- Sustainable crop feeds, fertilisers, pesticides and herbicides (or alternatives) - short term (2020 to 2025)
- Reduce energy consumption in agricultural production by low carbon machinery, minimum till cultivations, alternative energy sources, etc - short to medium term (2020 to 2030)
- Improved ground based and remote sensing technologies and modelling for robust carbon storage calculations for agricultural land - short to longer term (2020 to 2030s and beyond)
- Research to improve understanding of maintenance of soil systems to deliver multiple functions and developing an improved and efficient soil microbiome - short to medium term (2020 to 2030)
- Research into land management to achieve best use of different types of land - short term (2020 to 2025)
- Advances in measuring and monitoring of emissions and environmental impacts from the whole agri-food supply chain including social and economic impacts - short to medium term (2020 to 2030)
- Advances in plant breeding (including through biotechnology) to increase productivity, resilience to climate change, and to minimise the use of chemicals - short to longer term (2020 to 2030s and beyond)
- Demonstrator farms or regions to understand changes at system level, for example, agroforestry, paludiculture, novel ruminant housing systems - short to medium term (2020 to 2030)
- Field and farm scale data collection and sensor development - short to longer term (2020 to 2030s and beyond)
- Understand economic and behavioural barriers to change and how these can be overcome to support farmers and land-managers transition to net-zero - short to medium term (2020 to 2030)
- Research and pilot green finance and new farm business models to enable adoption and innovation of sustainable farming practices - short term (2020 to 2025)
Sustainable consumption:
- Development of models to rapidly reflect changes in food demand - short term (2020 to 2025)
- Develop metrics which can monitor and communicate embedded emissions in consumer goods - short term (2020 to 2025)
- Continue research to better understand the health, environmental and resilience impacts of changing end-user preferences and markets - short term (2020 to 2025)
- Research into social drivers of consumption and wastage and mechanisms to incentivise change - short term (2020 to 2025)
Developing a sustainable bioeconomy:
- Forestry, second generation energy crops, agricultural residues, wastes and novel feedstocks - short to medium term (2020 to 2030)
- Breeding to increase varieties and improve feedstock quality - short to medium term (2020 to 2030)
- Better GHG lifecycle assessments of the production of different types of biomass feedstock and means to reduce these impacts, such as the use of bioenergy with carbon capture and storage (BECCS) - short term (2020 to 2025)
- Understand locational / regional impacts on environmental services - short to medium term (2020 to 2030)
- Understand and mitigate wider sustainability impacts, such as air quality - short to medium term (2020 to 2030)
- Develop understanding of where bio-derived chemicals can displace fossil in the medium term; supporting these supply chains - short to medium term (2020 to 2030)
Waste and F-gases
Reducing waste and minimising emissions:
- Research anaerobic digestion (AD) technology: research ammonia emissions mitigation technology and wider impacts of digestate - short to medium term (2020 to 2030)
- Research into improved and more efficient AD - short to medium term (2020 to 2030)
- In depth review of landfill waste codes and their sources to allow targeted policies for biodegradable waste streams, with particular focus on commercial waste streams and construction and demolition waste - short term (2020 to 2025)
- Improved measurement of landfill emissions to allow a move away from estimates to empirical data, allowing capture of all landfill site-specific improvements and targetted interventions to reduce emissions at worst performing sites - short term (2020 to 2025)
- Investigate relationship between woodlanding and soil oxidation of methane, to free up more land to meet tree-planting targets, while simultaneously reducing landfill emissions through bio-covers - short term (2020 to 2025)
- Research policy options around reducing emissions from biological waste treatment, establishing technological, behavioural and economic barriers. This could also have impacts in the agriculture and wastewater sectors of the inventory, and provide better evidence for future bioenergy strategies - short term (2020 to 2025)
- Improve understanding of carbon impacts of waste prevention, resource efficiency, waste treatment and biodegradable waste policies - short term (2020 to 2025)
- Research technological, behavioural and economic barriers to reducing plastic use, and increasing recycling (‘reduce, reuse, repair, recycle’), including support for the development of bio-based and genuinely biodegradable plastics - short term (2020 to 2025)
- Research into optimising food production, quality, shelf life, improving resource use efficiency and influencing end-user choices to reduce food waste and waste associated with production, processing and across the supply chain - short term (2020 to 2025)
Reducing process emissions and energy use in the wastewater treatment sector:
- Research and modelling to deliver emissions savings from the wastewater component (particularly industrial) of the UK inventory through data improvements - short term (2020 to 2025)
- Research and roll out of widespread monitoring of emissions from wastewater treatment plants, aimed at optimisation of processes to minimise fugitive GHG emissions - short to medium term (2020 to 2030)
- Research and trials to understand and enable commecial deployment of novel treatment processes, including anaerobic, Membrane Aerated Biofilm Reactor and alternative processes for ammonia removal - short to medium term (2020 to 2030)
- Research into the barriers and implications of upgrading the UK water sector to advanced digestion processes - short to medium term (2020 to 2030)
- Research and options appraisal into the costs and benefits of different mitigation options available in the wastewater system, including through new technologies and changes to data gathering - short to medium term (2020 to 2030)
Minimising UK F-gas emissions:
- Research into the technical feasibility and cost-effectiveness of low-Global Warming Potential F-gases and alternatives to F-gases, with consideration of their energy efficiency and domestic and international standards and building and safety rules relevant to their uptake - short to medium term (2020 to 2030)
Enhancing the global coverage of atmospheric monitoring of Montreal Protocol controlled substances:
- Research to improve global coverage of atmospheric monitoring of ozone depleting substances and hydrochloroflourocarbons as Montreal Protocol controlled substances, including identifying gaps in global coverage of atmospheric monitoring and suitable locations for new monitoring sites. Also, piloting collaborative efforts to enhance monitoring of controlled substances - short to medium term (2020 to 2030)
5. An integrated Net Zero Research & Innovation Framework
What research and innovation is needed?
This Framework highlights that urgent research and innovation is needed to accelerate the commercialisation of solutions at scale, which can provide the carbon emission reductions needed in the UK for Carbon Budget 6 and for net zero. Larger-scale demonstrations and trials will need an increased share of research and innovation spending through the 2020s and 2030s, recognising that almost half of the CO2 reductions required to reach net zero by 2050 will need to come from technologies that are still in the prototype phase. Figure 24 illustrates key research and innovation challenges for the UK’s transition to net zero by 2050.
Renewable energy generation will need to be rapidly deployed with research and innovation driving continual improvements and unlocking new opportunities, such as floating offshore wind, as well as enabling reliable low carbon electricity through nuclear. Innovation will be needed to transform the wider electricity system and networks and to realise the potential for biomass across a number of hard to decarbonise sectors and through BECCS to drive negative emissions.
Continuing innovation to improve resource and energy efficiency alongside proving the feasibility of low and zero-carbon fuels and feedstocks (such as electricity, hydrogen and biomass) will be needed to decarbonise industry with residual emissions captured at source or offset by greenhouse gas removals. Hydrogen is expected to become a key energy vector with research and innovation needed to support the scale-up of low carbon hydrogen supply, demand, transportation and storage.
Even in ambitious decarbonisation scenarios, there are likely to be residual UK GHG emissions in 2050. Research and innovation is needed both to support an urgent deployment of industrial-scale CCUS technologies and to develop other GGR solutions to address those residual emissions.
In our homes and buildings, innovation is needed in technologies, processes, business models and consumer behaviour to support large-scale retrofit of low carbon heating and energy efficiency measures, and to support key decisions amongst candidate options for heating our homes.
On roads, electrification is expected to be the principal option but other low carbon fuels, particularly hydrogen, may have an important role to play, for example in HGVs, with research and innovation on refuelling and recharging infrastructure also of importance. Zero carbon solutions for aviation and maritime are still at much earlier stages of development and will require significant investment out to 2050. Research is also needed to understand how peoples’ journeys are changing and on solutions tailored for different local contexts.
How we best use the UK’s natural resources and land remains a key research question. The answer will require deep understanding of tensions and trade-offs between food production, forestry and biomass production, habitat and peatland restoration, urban expansion, and promoting greater carbon sequestration alongside wider environmental benefits. Scaling-up a sustainable bioeconomy, decarbonising the agricultural sector, tackling methane from waste and developing sustainable alternatives to F-gases will also require research.
Underpinning all of this is the need for a whole systems approach. Research will help to build our understanding of the interrelated nature of different sectors and between new technologies, consumer behaviour and business models. This lens helps to highlight key cross-cutting themes such as the integration of digital solutions across all parts of our economy and society to drive greater resource and energy efficiency. Above all the need for broad public support and development of viable markets and supply chains needs to go hand in hand with the development of new technologies if research and innovation is to help deliver our net zero ambition. Choices will need to be made and the solutions will need to be deployed at scale and at a sustainable cost.
Figure 24: Net Zero Research & Innovation Roadmap for UK
4.1 Power
2020s
- Innovation to accelerate the deployment of fixed offshore wind capacity and unlock deep water floating offshore wind (Development TRLs 4-6 & Demonstration TRLs 6-9)
- Innovation to prepare networks, demonstrating flexible demand and flexible market platforms, and developing long-term storage solutions (Development TRLs 4-6 & Demonstration TRLs 6-9)
- Improve biomass production and pre-processing; develop flexible gasification systems (various feedstocks, various end products); explore routes to deploy BECCS (Development TRLs 4-6 & Demonstration TRLs 6-9)
2030s
- Continue to identify the most cost effective and GHG-optimal approaches for biomass
2020s to 2030s
- Develop and deploy small modular reactors (SMRs) and demonstrate advanced nuclear reactors (AMRs) for electricity generation, hydrogen, and heat (Development TRLs 4-6 & Demonstration TRLs 6-9)
4.2 Industry
2020s
- Continuing energy and resource efficiency improvements, especially in heavy industries such as chemicals, cement, steel, and glass manufacture (Development TRLs 4-6 & Demonstration TRLs 6-9)
- Develop and demonstrate electrification, hydrogen and bioenergy, identifying which solutions are best suited to different industries (Development TRLs 4-6 & Demonstration TRLs 6-9)
- First-of-a-kind CCUS demonstration plants across industrial sources (Demonstration TRLs 6-9)
2030s
- Develop and demonstrate other low carbon fuels including process heat, for example from advanced nuclear reactors; support deployment of net zero industrial cluster by 2040 (Research TRLs 1-3, Development TRLs 4-6 & Demonstration TRLs 6-9)
- Scale-up of CCUS, including innovation in alternative means of transport and storage for dispersed sites
2040s
- Last mile issues to ensure almost no unabated fossil fuel in use in industry by 2050 (unless combined with CCUS) (Research TRLs 1-3, Development TRLs 4-6 & Demonstration TRLs 6-9)
4.2 Hydrogen
2020s
- Demonstrate efficient CCUS-enabled hydrogen and scale-up to large production capacity in industrial clusters (5GW ambition by 2030) (Demonstration TRLs 6-9)
- Develop and demonstrate hydrogen production via electrolysis at different sites; develop lower TRL production techs, such as BECCS, nuclear (Research TRLs 1-3, Development TRLs 4-6 & Demonstration TRLs 6-9)
- Demonstrate effective, low-cost methods of bulk hydrogen storage and transportation (Demonstration TRLs 6-9)
2030s
- Research to support deployment of increased hydrogen production capacity, enabling of supply chain development, and transitioning from CCUS-enabled hydrogen to electrolytic hydrogen
- Demonstrate longer distance hydrogen transmission, scaling up to international transport in 2030s; new, more efficient options for storage (Development TRLs 4-6 & Demonstration TRLs 6-9)
4.3 CCUS & GGRs
2020s
- Demonstration of CCUS, including industry and hydrogen production; initial demonstration of BECCS and gas with CCUS; improvements to capture rates and efficieny (Development TRLs 4-6 & Demonstration TRLs 6-9)
- Innovation to develop supply chains, including on business models, financing, and risk sharing arrangements (Demonstration TRLs 6-9)
- Research to identify lowest-cost transport infrastructure opportunities; de-risking scale-up of CO2 stores; improving measuring, monitoring and verification (Research TRLs 1-3 & Development TRLs 4-6)
- Develop greenhouse gas removal technologies and nature-based solutions (Research TRLs 1-3, Development TRLs 4-6 & Demonstration TRLs 6-9)
2030s
- Research to support large-scale deployment of CCUS for industry and hydrogen production; scaling-up demonstration of BECCS and gas with CCUS (Development TRLs 4-6 & Demonstration TRLs 6-9)
- Innovation for development of offshore storage and for alternative means of transport, storage and capture technology at dispersed sites
- Demonstrate DACCS and other GGR approaches at scale
2040s
- Improve efficiency and reduce energy demand of GGRs; research to support deployment of GGRs to meet targets
4.4 Heat and Buildings
2020s
- Test whole house/building retrofit for domestic and non-domestic; develop new supply chain models; energy efficiency solutions for hard-to-treat properties (Demonstration TRLs 6-9)
- Demonstrate safety and feasibility of hydrogen heating, increase readiness of appliances and consumer trials. Decision on hydrogen for heating (by 2026) (Development TRLs 4-6 & Demonstration TRLs 6-9)
- Reduce costs of heat pump manufacture; reduce disruption from installation and use; develop innovative business models to drive uptake; demonstrate in range of homes and at neighbourhood/district scale (Development TRLs 4-6 & Demonstration TRLs 6-9)
2020s to 2030s
- Research ways to incentivise connections to heat & cooling networks; develop innovative solutions to access heat from existing and future heat sources (Research TRLs 1-3, Development TRLs 4-6 & Demonstration TRLs 6-9)
4.5 Transport
2020s
- Improve the efficiency of EV manufacture; trialling infrastructure improvements including charging, vehicle to grid, flexible/smart charging; novel battery technology (Development TRLs 4-6 & Demonstration TRLs 6-9)
- Research deployment options for hydrogen and electric buses; demonstration of HGV technologies including hydrogen, battery, electric road systems; mega-charging for HGVs (Research TRLs 1-3, Development TRLs 4-6 & Demonstration TRLs 6-9)
- Demonstrate hydrogen trains and infrastructure; research hydrogen distribution by rail to support other sources of demand (Research TRLs 1-3, Development TRLs 4-6 & Demonstration TRLs 6-9)
- Develop zero emissions aircraft and airside vehicles; prepare UK airports for zero emission aircraft; facilitate UK R&D on aerospace manufacturing (Research TRLs 1-3 & Development TRLs 4-6)
2020s to 2030s
- Demonstrate zero emission vessels, including with batteries, hydrogen, and ammonia; hydrogen and ammonia bunkering and dispensing at ports; zero emissions ports (Development TRLs 4-6 & Demonstration TRLs 6-9)
2030s to 2040s
- Continued development of more efficient aircraft; certification and infrastructure requirements; develop and trial sustainable aviation fuel manufacture
4.6 Natural resources and land-use
2020s
- Research biomass supply including forestry, energy crops, agriculture residue, waste and novel feedstocks; improved GHG lifecycle assessments; crop breeding (Research TRLs 1-3)
- Research for anaerobic digestion and ammonia emission mitigation; food production and consumer behaviour to reduce food waste; trial bio covers on landfill (Research TRLs 1-3)
- Research to develop tools to inform land-use decisions; research to understand interplay between actors, policy/regulatory framework, incentives and technologies (Research TRLs 1-3, Development TRLs 4-6 & Demonstration TRLs 6-9)
- Research coastal wetlands, including scale and direction of change in carbon storage; design of offshore infrastructure; sustainable salt and fresh-water aquaculture systems (Research TRLs 1-3)
2020s to 2030s
- Research for sustainably expanding and managing forests, including improved tech and modelling for carbon storage; research on woodland types and silviculture; innovative finance models; social/behavioural drivers and barriers (Research TRLs 1-3, Development TRLs 4-6 & Demonstration TRLs 6-9)
- Research into lowland peat management with farming; improved GHG assessment of peatland restoration; optimal water management for emissions & flood risk (Research TRLs 1-3, Development TRLs 4-6 & Demonstration TRLs 6-9)
2020s to 2040s
- New practices and technologies to reduce agricultural emissions including precision farming; animal/crop breeding; sustainable fertilisers and pesticides; economic and behavioural barriers to change; demonstrator farms (Research TRLs 1-3, Development TRLs 4-6 & Demonstration TRLs 6-9)
3. Systems-wide/ cross-cutting
2020s to 2040s
- Understand optimal net-zero pathways, interdependencies and trade-offs across physical, natural, social and technological systems (Research TRLs 1-3 & Development TRLs 4-6)
- Integrate changes to energy supply, storage and use with increasing interlinkages between energy vectors and across different sectors of the economy (Research TRLs 1-3, Development TRLs 4-6 & Demonstration TRLs 6-9)
- Enable an integrated, multi-modal transport system and accelerate the adoption of active travel and public transport; understand role of transport in the wider energy system, including the hydrogen economy (Research TRLs 1-3, Development TRLs 4-6 & Demonstration TRLs 6-9)
- Digital solutions and technologies to support cross-sector integration, enable systems-level understanding and unlock resource and energy efficiency (Research TRLs 1-3 & Development TRLs 4-6)
- Beyond technology, there is a need to understand how more sustainable behaviours can be incentivised, to develop new types of business and financial models and to deliver locally and regionally appropriate solutions (Research TRLs 1-3 & Development TRLs 4-6)
How to prioritise public investment?
The Net Zero Strategy sets out several viable pathways to net zero and we should avoid locking ourselves into any one technology pathway prematurely. This means investing widely and accepting some investments will not deliver the expected benefits or offer a viable commercial proposition. Ultimately it is the commercialisation of innovative low-carbon products and services, not the innovation itself, that leads to benefits by way of lower energy costs, reduced emissions of greenhouse gases, energy security and business growth.
However, within this we will need to prioritise. Working back from 2050, Figure 24 shows the sequence for technologies (and research for policy decisions) as they move through early-stage research, feasibility and technical development and onto demonstration at scale, to reach commercial deployment in time to deliver our Net Zero commitment.
For government investment, we will prioritise spend based on:
- Expected contribution to delivering the UK’s carbon budgets and major decarbonisation – accelerating delivery of carbon emissions reductions by increasing certainty of technologies / solutions, including by taking into account the current state of technologies and the potential for research and innovation to make rapid progress.
- Building and using UK comparative advantage globally – focussing on areas with the highest potential for UK business and jobs. Developing and commercialising technologies, processes and business models for the net zero transition can provide business opportunities and enhance economic competitiveness.
- Retaining optionality of different net zero pathways – investing in a portfolio of solutions (tolerating some failure) and ensuring technologies which we cannot reach net zero without are invested in, including greenhouse gas removals.
Government intervention will be needed where market failures prevent private sector investment, to de-risk and accelerate private sector action or where there is potential to develop areas of UK strategic advantage. This public research and innovation spending can be used to push technology development, which will complement market-pull mechanisms such as policy, regulatory and financial frameworks (for example, Contracts for Difference; Internal Combustion Engine phase-out). Both approaches will be needed for success.
Based on our current understanding we expect to prioritise:
Major decarbonisation opportunities
- Floating offshore wind
- Energy storage at scale and system flexibility - enablers of high renewables system
- Hydrogen - enabler of industrial fuel switching, heat and some negative emissions
- Carbon capture, utilisation and storage for industry - critical for hard to abate areas
- Buildings decarbonisation
- Land transport, including zero emission road vehicles, rail, light rail and active travel
- Aviation and maritime
- Agriculture and food
- Nature-based carbon removals, for example afforestation, domestic perennial energy crops, short rotation forestry, and biochar.
Major business opportunities
- Transport - aviation, automotive, maritime
- Energy storage at scale
- Hydrogen
- Nuclear - Small Modular Reactors, Advanced Modular Reactors and advanced fuel cycle, particularly in export
- Offshore wind - with floating offshore wind potential new area for export and domestic deployment
Creates optionality in net zero pathways
- Energy efficiency
- Carbon capture, utilisation and storage - major enabler for industry, hydrogen and bioenegry with carbon capture and storage (BECCS)
- Innovation within industrial energy sectors - hard to abate and cannot be substituted by other technologies
- Sustainable land-use
- Negative emissions technologies including Direct Air Capture
How should this Framework be used?
This Framework lays the foundation for net zero research and innovation planning within government and aims to provide innovative businesses and academic and research communities with a tool to guide their own research and innovation agendas. We do not expect government programmes to cover all the needs identified by this Framework and we will prioritise those where government intervention is most needed based on the criteria outlined above. As research delivers more answers it should be possible to focus on scale-up and deployment spend in the key areas.
For publicly funded research and innovation, the government intends to publish a Delivery Plan to show what current and planned programmes are being prioritised from this framework. The Net Zero Innovation Board[footnote 47], chaired by the Government Chief Scientific Adviser, provides the main government forum for discussing prioritisation and spending plans related to net zero research and innovation and will play a key role in assessing progress against the Delivery Plan and this wider Framework.
Given the scale of the transformation needed to reach Net Zero by 2050 any framework will necessarily need to adapt and change over time. We cannot, nor would we want to, plan for all the research and innovation needs over the next 30 years. Breakthrough technologies could revolutionise our understanding and approaches in some areas. Government, as well as business, will need to be agile and able to pivot our plans in the future. However, by publishing the UK’s first Net Zero Research and Innovation Framework, we hope to provide fresh impetus to those goals, in the next 5 to 10 years, where it is known that research and innovation is urgently needed and drawing a line of sight from these out to 2050. A detailed plan for delivery against this Framework will follow.
Endnotes
-
Chapters have been defined based on the underlying research and innovation challenges. In particular, hydrogen production has been highlighted within the industry chapter and end-uses of hydrogen within their related sector chapter. Similarly, given CCUS’s potential impact across the net zero system, it has been highlighted as an area of focus alongside greenhouse gas removal (GGRs) technologies. ↩
-
In the International Energy Agency’s 2050 net zero scenario, 46% of the annual CO2 savings come from technologies still under development: IEA (2021) Net Zero by 2050 ↩
-
Energy Innovation Needs Assessment. BEIS/Vivid Economics (2019). Figures based on a 80% reduction in emissions by 2050. ↩
-
Internal BEIS analysis based on the Energy Innovation Needs Assessment ↩
-
UK Board of Trade Report (July 2021) ↩
-
Clean Growth Strategy (2018) ↩
-
IEA data (2019) ↩
-
IEA (2021) Net Zero by 2050 ↩
-
TechNation (2020) UK Tech For A Changing World ↩
-
See Committee on Climate Change (2021) Independent Assessment of UK Climate Risk for additional information on risks to net zero from climate change ↩
-
Mission Innovation (2021) ↩
-
BEIS (2020) ↩
-
HM Government (2020) Energy White Paper ↩
-
HM Government (2020) Energy White Paper ↩
-
Mission Innovation (2021) ↩
-
BEIS and Ofgem (2021). Transitioning to a net zero energy system: smart systems and flexibility plan 2021 ↩
-
BEIS (2020). Modelling 2050 – electricity system analysis. Quoted in 2012 prices, undiscounted ↩
-
Digest of UK Energy Statistics (2021) Chapter 1: Energy ↩
-
See Climate Change Committee (2018) Biomass in a low carbon economy ↩
-
ONS, 2021 – Gross Domestic Product ↩
-
UK in a Changing Europe, 2020 – Manufacturing and Brexit ↩
-
This figure relates to industry sectors covered by the Industrial Decarbonisation Strategy (2021) and excludes related power sector emissions ↩
-
IEA (2021) Net Zero by 2050 ↩
-
Pathways modelled by BEIS for the 6th carbon budget impact assessment show that 250 – 460 TWh of hydrogen could be needed by 2050. Further analysis that draws on a number of sources indicates a range of 125 – 495 TWh. Throughout the 2030s and 2040s hydrogen demand is expected to rapidly increase, requiring hydrogen production capacity to increase from 5 GW in 2030 to 7 – 20 GW in 2035 and 15 – 60 GW in 2050. ↩
-
Hydrogen Strategy (2021) ↩
-
Hydrogen Strategy (2021) ↩
-
The Geological Society (2021) ↩
-
Mission Innovation (2021) ↩
-
The Ten Point Plan for a Green Industrial Revolution (2020) ↩
-
Net Zero Strategy (2021) ↩
-
National Statistics (2020) Households projections for England. BEIS (2020) Non-domestic National Energy Efficiency Data-Framework ↩
-
BEIS (2021) Final UK greenhouse gas emissions national statistics: 1990 to 2019 and BEIS (2021) Energy Consumption in the UK. ↩
-
MHCLG (2020), English Housing Survey 2019 to 2020: headline report Annex Table 2.8. ↩
-
Approximately 28 million homes and 2 million non-residential buildings. National Statistics (2020), Households projections for England, Table 401; BEIS (2020), ‘Non-domestic National Energy Efficiency Data-Framework’, based on 2018 data ↩
-
For example, the Energy White Paper estimates that bringing 2.8 million privately rented homes to EPC rating C could create 80,000 jobs per year, and installing 600,000 heat pumps a year could support 20,000 jobs by 2030 ↩
-
BEIS analysis based on the Energy Innovation Needs Assessment (Vivid Economics 2019) ↩
-
Net Zero Strategy (2021) ↩
-
Mission Innovation (2021) ↩
-
Climate Change Committee (2020) Sixth Carbon Budget Report (includes adjustments from the UK’s national inventory) ↩
-
Climate Change Committee (2019) Net Zero Technical Report ↩
-
Climate Change Committee (2019) Net Zero Technical Report ↩
-
See: Climate Change Committee (2020) Sixth Carbon Budget Report. Globally, food systems - including agriculture, manufacturing, processing, packaging and consumption are estimated to be responsible for around one third of global GHG emissions. ↩
-
Climate Change Committee (2019) Net Zero Technical Report ↩
-
Net Zero Innovation Board (2021) ↩