Aerosol and droplet generation from singing, wind instruments and performance activities, 13 August 2020
Updated 1 December 2021
Singing, Wind Instruments (SWI) and Performance Activities Working Group Consensus Statement
Singing, playing of wind instruments, and high-volume speech in presentation and performance settings have been singled out as potentially high-risk activities for transmission of SARS-CoV-2, following several well-documented outbreaks associated with choirs and performances across the world. These have raised questions about the potential for droplet and aerosol transmission from these sources.
We have reviewed the international evidence base and commissioned 2 research trials (PERFORM and SOBADRA) to investigate droplet and aerosol production in performance events. Using the knowledge obtained through these scientific enquiries, we have considered the real-world implications for the management of these activities to minimise the risks associated with them. This work is on-going and we outline recommendations for further research and analysis.
1. Evidence base
1.1. The international evidence base has previously been collated in NERVTAG papers on a) the risks of transmission in singing events and b) the role of aerosol transmission in COVID-19.
1.2. The evidence base continues to develop during the pandemic and the literature has expanded since these papers were submitted.
1.3. A major study (The International Performing Arts Aerosol Study) is ongoing, led by the University of Colorado Boulder. Preliminary recommendations from the first phase of this study (woodwind and brass) include the wearing of adapted surgical masks (with slits), increased distancing for trombones, and nylon bell covers for brass instruments. Recommendations are also made on rehearsal duration and rehearsal spaces. However, preliminary reports of the study do not contain enough quantitative data for meaningful analysis and comparison.
1.4. A recent study from Berlin (Murbe et al.) identified significantly higher aerosol emission rates for loud singing compared to breathing or speaking. This examined a small number of individuals, did not explore loudness of speaking and there was a large variation in aerosol emission.
2. Summary of key findings from PERFORM and SOBADRA
2.1. Droplets refer to respiratory particles that rapidly deposit onto surfaces. These are measured through detecting deposition of particles onto water sensitive paper or through microorganisms that settle on petri dishes. Social distancing is a primary mitigation measure for minimising the risk of droplet transmission.
2.2. Aerosols refer to respiratory particles, typically less than 10 µm in diameter, that can remain airborne for long periods of time. These are measured using aerosol particle counters or bioaerosol samplers. Ventilation and social distancing are important mitigation measures for minimising the risk of aerosol transmission. Aerosol risk is also higher closer to the source, particularly in an indoor environment.
2.3. It should be noted that these studies do not measure virus. The studies use aerosol particles and/or oral bacteria as proxy markers for the potential for virus to be dispersed in droplets and aerosols. The transmission risk is assumed to be related to the amount (mass) of droplets and aerosol that is generated, but this cannot be confirmed currently.
3. Risks posed by droplets
3.1. The total mass of droplets generated from singing is a similar order of magnitude to speaking at a comparable volume for the same time duration. (high confidence level).
3.2. Droplet deposition onto surfaces from singing and speech does not generally extend beyond 2 metres from the subject (high confidence level).
3.3. No greater than 40µm droplet production was identified from 12 wind and brass instruments studied (high confidence level).
3.4. Oral bacteria can be detected in droplets and aerosols generated during respiratory activities, including singing. This shows that droplets can carry microorganisms.
3.5. Significantly fewer oral bacteria are dispersed during singing and speech than during more ‘dramatic’ respiratory activities (for example coughing) (medium confidence level).
3.6. The variance in droplet generation and dispersion of oral bacteria between individuals is high (high confidence level).
4. Risks posed by aerosols
4.1. Breathing, speaking and singing all produce aerosol (high confidence level).
4.2. Regular conversational speaking produces approximately the same amount of aerosol (in terms of total mass across all particle sizes, referred to simply as ‘aerosol mass’ below) as breathing (high confidence level).
4.3. Singing produces more aerosols (a statistically significant factor of between 1.5 to 3.4 in median number and mass concentration) than speaking at a similar loudness (medium confidence level).
4.4. The loudness of singing and speaking is a significant factor in determining the amount (total mass) of aerosol generated:
4.4.1. Singing and speaking at a low or medium loudness does not produce significantly more aerosol than breathing (medium confidence level).
4.4.2. Very loud singing and speaking can generate around 20 to 30 times more aerosol (in terms of total mass) than breathing, quiet singing and speaking (high confidence level).
4.5. Some individuals produce a much greater mass of droplets and aerosol than other people, to the extent that breathing from a small number of people (2 out of 25 in the PERFORM study) generates as much material as singing at the loudest volume does by others. (high confidence level).
4.6. Further analysis of wind and brass instruments is awaited.
4.7. Clearing spittle or condensation from brass instruments does not produce aerosol particles. (high confidence level).
5. Real world implications of findings
5.1. The SARS-CoV-2 virus can only be transmitted in a performance setting if there are infectious individuals present amongst the audience or performers. All relevant measures outlined in current Performing Arts Guidance that are required to make an event or performance setting COVID-Secure should continue to be applied.
5.2. In terms of droplet spread, social distancing is a prime mitigation. In terms of aerosol, social distancing and ventilation are important mitigation measures.
5.2.1. Since singing and speaking are broadly similar in terms of droplet production, extended social distancing (greater than 2 metres) to mitigate droplet transmission by singers does not seem necessary (high confidence). There is a remote chance of droplet transmission at a social distance of 2 metres.
5.2.2. The significance of extended social distancing (greater than 2 metres) to mitigate aerosol transmission by singers (singing generates an increase of 1.5 to 3.4 time more aerosol mass than speaking) is not known. It is likely that beyond 2 metres aerosol transmission risk will be determined by the room airflow pattern.
5.2.3. Since droplet spread from wind and brass instruments is negligible, extended social distancing (greater than 2 metres) to mitigate droplet transmission is not necessary (high confidence). Normal social distancing is still required as performers can produce aerosols and droplets when not playing the instrument. There is a remote chance of droplet transmission at a social distance of 2 metres.
5.3. Breathing, speaking and singing all produce aerosol; the contribution of potential aerosol contamination from audience and performers must be considered together. As singing at high volume can generate 20 to 30 more aerosol than breathing or quiet speaking or singing, ventilation provision should be enhanced to effectively dilute or remove these higher levels of aerosol. The enhanced ventilation will need to consider the duration of singing or loud speech during a particular performance or event. Ventilation provision for singers on a per performer basis may therefore need to be up to 20 times that for audience members for the equivalent period during which they are actually singing.
5.4. Building on existing guidance, further recommendations should be based on the loudness, amplification and duration of the activity, the number of performers, the vulnerability of the performers and the audience, the size of the audience, the environment in which the activity occurs and the background prevalence of SARS-CoV-2.
5.4.1. Small venues are likely to pose a significant challenge. Groups who use such venues should maximise ventilation and limit both numbers of people and the duration of activities. Where possible groups are advised to perform in larger spaces if these are available.
5.4.2. All singing and dramatic performances should consider limiting the number of performers and audience members, using larger halls or rooms, limiting the length of the activity, using microphones, and providing fresh air at a rate of at least 10 litre per second per person. The rate of 10 litre per second per person reflects building regulations for offices rather than a result of this study so confidence in this figure is lower; the higher the ventilation rate the better. These measures will make transmission unlikely.
5.5. Socially-distanced, outdoor performances of singing or wind and brass instruments present a low risk of droplet or aerosol transmission. Transmission of SARS-CoV-2 is highly unlikely if the space is fully open.
5.6. Analysis has been carried out predominantly for professional singers and musicians. There is no known reason why there should be a substantial difference in aerosol and droplet generation between amateur and professional musicians; however, the vulnerabilities between these groups may vary.
6. Recommendations for further research and analysis
6.1. Data from these studies should be used in risk models to evaluate relative risks in different performance and setting scenarios. Appendix 3 presents a simple model, but further work is necessary to better characterise risks.
6.2. The aerosol generation data to date has been for a limited number of instruments and mostly professional singers. Analysis of further data on aerosol generation from a wider range of wind instruments, amateur singers and a broader range of singing styles is important for completeness of the study.
6.3. The studies to date have included a small number of participants (25 PERFORM, 12 SOBADRA) and both have identified significant variability in the aerosol and droplet generation between participants. A small number of participants (2 out of 25) generated as much aerosol and droplet whilst breathing as others did from singing at the loudest volume. It is important to conduct further measurements with a greater number of people to determine how significant this variation is within the population.
6.4. All the data in this study is from adult participants. Data on aerosol generation by children would be important to understand potential risks in school and youth activities
6.5. Analysis is based on measurement of inert droplets and aerosols and oral bacteria. Research to relate this data to viral aerosol generation is challenging, but important to fully characterise risks.
6.6. Aerosol generation is identified as likely posing an important risk. Research to fully understand the risks this poses in different performance venues, including details of the mitigation provided by ventilation and the influence of spatial variation in airflow patterns created by occupants and the building systems, is a high priority. This should include experimental and computational simulation models coupled to airborne transmission risk models.
Appendix 1: PERFORM Study
Study setting:
The study was performed in 2 orthopaedic operating theatres in central London. The high air exchange rate, filtration and laminar air flow reduced the pre-existing particle background number concentration over the measured size range to 0 centimetres to 3, enabling extremely sensitive measurements using an array of standard aerosol measurement instrumentation (particularly aerodynamic particle sizers) and water sensitive paper to measure droplet production.
Study participants:
The cohort of 25 professional singers perform a broad range of genres, including:
- musical theatre (6)
- choral (5)
- opera (5)
and other genres:
- gospel (2)
- rock (2)
- jazz (2)
- pop (1)
- actor with singing interest (1); and
- soul (1)
6 identified their voice-type as soprano or mezzo-soprano, 7 as alto, 5 as tenor and 7 as bass or baritone. Woodwind and brass instruments were also studied, specifically: trumpet, trombone, French horn, tuba, flute (with 3 performers), piccolo, clarinet, saxophone, oboe and bassoon.
Study objectives:
Our objective were:
- To directly measure aerosol production rates from singing, speaking, breathing, coughing and playing instruments in a zero-background environment from professional musicians and amateur singers, allowing unequivocal attribution of aerosol production to specific vocalisations or instrument performance.
- To provide a robust analysis that allows the aerosol mass concentrations and size distributions from these different activities to be direct compared; and
- To assess large droplet production from these activities.
The results of Phase 1 of the PERFORM study have been written-up as a pre-print manuscript and have been lodged in the evidence repository:
‘Comparing the Respirable Aerosol Concentrations and Particle Size Distributions Generated by Singing, Speaking and Breathing. Reid et al.’
Appendix 2: Sampling for oral bacteria in aerosols and droplets from respiratory activities (SOBADRA): a study to inform risk assessment of transmission of SARS-CoV-2 infection
Study setting:
The study was conducted at PHE Porton within a small test chamber (4 metres times 2.5 metres times 2 metres) supplied with HEPA filtered air. Venting the toom (2 air changes a minute) between experimentsensured a low level of background aerosol.
Study participants:
Ten amateur singers (PHE Porton staff; 5 female) and 2 professional choristers (bass; alto) from Salisbury Cathedral volunteered to take part in the study. Each volunteer wore a Tyvek suit, gloves and overshoes and was asked to perform a series of expiratory activities (count (1 to 100), cough and exhale through the mouth or nose) and to sing ‘O Come All Ye Faithful’. The two choristers were also asked to recite ‘O Come All Ye Faithful’ and to sing one or more songs of their choosing.
Study objectives:
1) To determine the type and concentration of oral (respiratory) bacteria dispersed by volunteers. 2) To assess the impact of singing on droplet and aerosol generation. 3) To investigate factors that could influence droplet and aerosol generation during singing.
Key findings
Saliva contains a high concentration of bacteria. The mean number of oral bacteria (OB) recovered from saliva (n = 10) was 7.2 times 107 colony forming unit (cfu) per mililetre. Predominant organisms were from the oral cavity (for example Streptococcus salivarius) and the upper respiratory tract (for example Neisseria subflava). A recent study reported less than 106 viable SARS-CoV-2 per ml of saliva (personal comms from University of Calgary to A Bennett).
OB can be detected in droplets and aerosols generated during respiratory activities, including singing.
Significantly fewer OB are dispersed during singing than during more ‘dramatic’ respiratory activities (for example coughing) and dispersion variance between individuals is high. Dispersal differed with individual. When performing a series of respiratory activities, only 3 out of 12 participants dispersed high numbers of respiratory droplets. The mean number of OB deposited on surfaces within 1 metre of these singers was 105 cfu (3 cfu/10 cm2). During singing, this number reduced 10-fold. The mean number of OB recovered from air samples taken 1 metre from the three participants reduced 8-fold, from 56 cfu/m3 to 7 cfu/m3 .
Singing does not generate higher concentrations of respiratory aerosols than talking but ‘voice’ may influence droplet dispersal. The mean number of OB recovered from air samples taken 1 metre in front of participants whilst they recited (68 to 73db) and sang (80 to 83db) the same song equated to 4 cfu per cubic metre per minute and 1 cfu per cubic metre per minute respectively (n = 3) suggesting no increased risk of aerosol transmission.
The number of OB deposited on surfaces within 1 metre of one ‘amateur’ singer (baritone) increased from 4 cfu (0.1 cfu/10 cm2) when speaking to 9 cfu (0.2 cfu/10 cm2) when singing. This increase was also captured by the Andersen air sampler. During singing more OB were collected on stages 1 to 3 (were associated with particles greater than 3.3 μm in diameter) than during recital of the same song. No OB associated with particles less than 3.3 μm were recovered. In contrast, no OB associated with particles greater than 1 μm were recovered from air samples taken 2 metre from the same participant. The professional choristers (alto; bass) did not generate more droplets during singing.
Song choice could increase short-range droplet dispersal. When singing the same carol, professional singers did not disperse more OB in droplets or aerosols than amateur singers. Singing English-language choral music did not increase bacterial dispersal but singing in German did increase the number of OB deposited immediately (0.2 m) in front of the singer from 1 cfu (0.1 cfu/10cm2) to 7 cfu (1 cfu per 10cm2). The concentration of aerosol did not increase.
Relevance to SARS-CoV-2. If a highlevel estimate of 1 times 106 viable SARS-CoV-2 per ml in saliva is used as an oral concentration and 7.2 times 107 per ml is the average OB concentration, then estimated levels of SARS-CoV-2 containing particles in the air would be 1.3% of those detected in these experiments. Using this relationship and the cohort of three disseminating singers, the estimated output of SARSCoV-2 would be 0.039 viral particles deposited per 10 cm2 and 0.728 viral particles/m3 of air during respiratory activities and 0.0039 viral particles deposited per 10 cm2 and 0.091 viral particles/m3 during singing.
Appendix 3: Evaluating risk of transmission with ventilation rate and activity.
The following presents results from a simple airborne transmission risk model to assess how the enhanced aerosol generation from singing or performance speech, the venue size and ventilation, and the occupancy density affect transmission risk.
Transmission is only considered by an airborne route as the model is concerned with aerosol generation. It is possible that droplet or surface transmission also poses a risk to those in close proximity to the infector.
Wells-Riley Risk Model
The Wells-Riley model is a well-known model used to relate risk of infection to the pathogen emission rate and the ventilation in a building. Under steady state contamination conditions a deterministic version of the model is gives the proportion of new infections as:

Here I is the number of infectors, S is the number of susceptible people, N is the number of new cases, p is the breathing rate (cubic metre per minute), t is the time (minute), Q is the removal (ventilation) rate (cubic metre per minute), and q is the quanta emission rate (quanta per minute) which is parameter that represents the rate of generation of infectious doses by the infector(s).
Under a typical performance scenario steady state contamination is not an appropriate assumption, as it would be expected that the space is free of viral load in the air at the beginning of the performance. Therefore an alternative transient formulation can be used which also considers the volume of the space, V (m3). This is given by:

Where
![Equation: A equals minus I qp dvided by Q [t minus V divided by Q (1 minus e times minusQt divided by V) ].](https://assets.publishing.service.gov.uk/government/uploads/system/uploads/attachment_data/file/1037719/formula-32.png)
While this accounts for transient variation in the airborne contamination, it does not consider spatial variation. The model presented here treats the performance venue as a single uniformly mixed space. This assumption is acceptable for smaller venues, but should be treated with caution for larger spaces where the airflow will not be uniformly distributed. It is possible to create more complex models that consider multiple zones including using computational fluid dynamics to simulate detailed airflows; it is recommended that this type of modelling is carried out.
Scenarios
Two scenarios have been modelled for 60 and 120 minute events with ventilation rates ranging from 1 litre per second per person to 100 litre per second per person (based on full venue occupancy) and the proportion of time singing or speaking from 0 to 75%. In each case risks are calculated for 1, 10 and 50 quanta per hour to represent a low, mid and high viral load. These are based on data in Buonanno et al 2020, however there is a high degree of uncertainty with these values , and in particular it is not clear what proportion of people are carrying a high viral load.
The effects of singing or performance are considered through increasing the quanta generation rate in line with the data from the PERFORM study. Loud singing is assumed to increase quanta generation by 20 times. A weighted average quanta emission rate is calculated by assuming different proportions of a performance time spent singing compared to breathing or quiet singing or speech. In each case one singer is assumed to be infected
Scenario | Size |
---|---|
Mid-sized theatre (250 seat) | 1.2 metres2 per seat + 1 metres2 per seat for aisles or stage = 550 metres2 with a 4 metre high ceiling |
Community venue such as a village hall | 18 times 10 metres = 180 metres2 with a 3 metre high ceiling |
Results
Model outputs in Figures 1 and 2 show viral load, time, venue size, proportion of time singing and ventilation rate are all likely to have an influence on the risk of transmission.
In a large venue, the risk of transmission remains below 1% when an infectious case has a low viral load regardless of the ventilation rate and proportion of singing, however in a small venue a two hour duration with singing over 50% of the time may push the risk beyond 1% for the lowest ventilation rates.
A mid viral load in the large venue requires only 6 litre per second per person to maintain a 1% risk in a 60 minute event at the highest duration of singing, but more than 10 litre per second per person in a 2 hour event with 50% singing. In a small venue over 10 litre per second per person is required to maintain a 1% risk in a 60 minute event with only 25% singing, while more than 20 litre per second per person would be needed for a 2 hour event. This level of ventilation may prove challenging for many smaller venues, particularly those that are naturally ventilated. The highest viral load proves challenging to control in both settings, with even breathing requiring at least 5 litre per second per person in a large venue for a 2 hour event. A small venue requires unfeasibly large amounts of ventilation to prevent transmission. The viral load in this case (small venue, 75% singing) is comparable to that calculated in the Skagit choir outbreak (Miller et al 2020 pre-print).
Although comparison here has been made by considering a 1% risk, it is important to recognise that the likely number of cases resulting from this depends on the number of people in the venue. The small venue with social distancing may only have 30 people present, while the larger venue could potentially have 100 plus people.
The models presented here assume that an infectious person is present in the venue, however in reality this will depend on the prevalence of the virus within the population. The risks presented here will be substantially reduced where there is lower prevalence of disease.
Implications and further research
All venues should maximise their ventilation rate as far as feasible, with at least 10 litre per second per person as a minimum target. Venue capacities should be limited to ensure this is achieved. Further enhanced ventilation should be provided where a performance involves sustained duration of loud singing or very loud speech.
Small venues are likely to pose a significant challenge. Groups who use such venues should maximise ventilation and limit both numbers of people and the duration of activities. Where possible groups would be advised to perform in larger spaces if these are available.
There is considerable uncertainty over the viral load, and how frequently high viral shedding is experienced. Further data is urgently needed on the variation in viral aerosol produced by different people. The likelihood of a high viral shedder will increase with community prevalence of the virus. The model presented here is a very simple deterministic risk model that does not consider spatial variation in airflow or risk. More complex models that incorporate multiple zones and stochastic effects, and computational fluid dynamics of airflows are recommended to understand transmission risks in a range of settings.
G. Buonanno, L. Stabile, and L. Morawska, “Estimation of airborne viral emission: Quanta emission rate of SARS-CoV-2 for infection risk assessment,” Environ. Int., vol. 141, no. April, p. 105794, 2020, doi: 10.1016/j.envint.2020.105794S.
S. L. Miller et al., “Transmission of SARS-CoV-2 by inhalation of respiratory aerosol in the Skagit Valley Chorale superspreading event,” medRxiv, no. June, p. 2020.06.15.20132027
Figure 1: Proportion infected with ventilation rate and percentage of singing in a mid-sized theatre
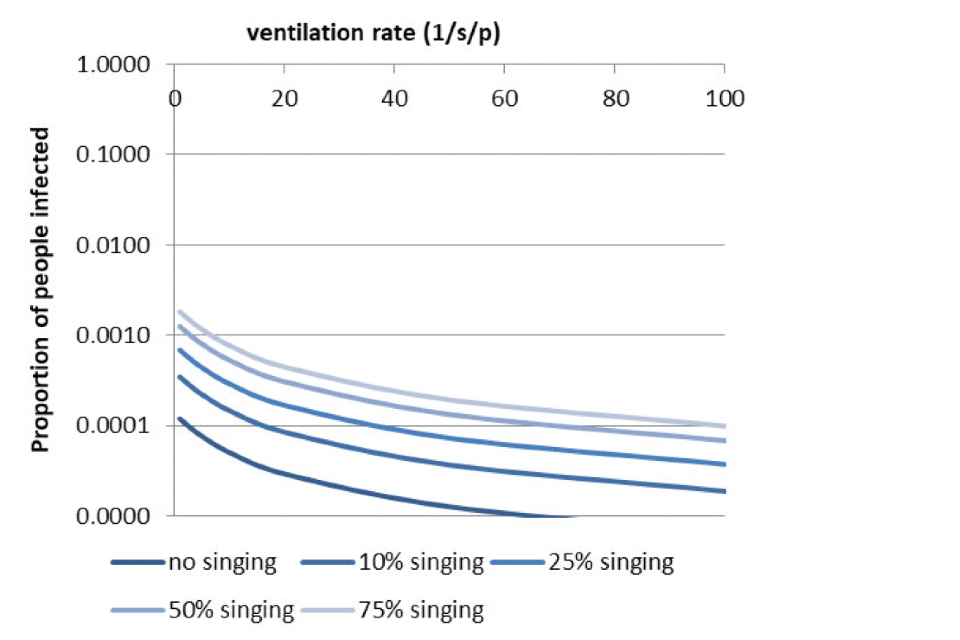
1 quanta per hour for a 60 minute event.
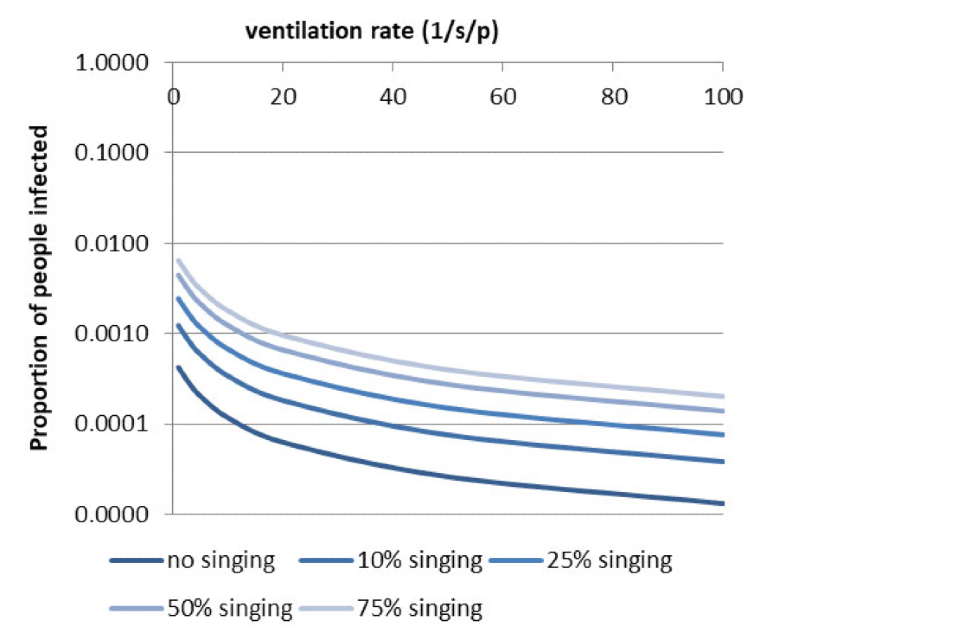
1 quanta per hour for a 120 minute event.
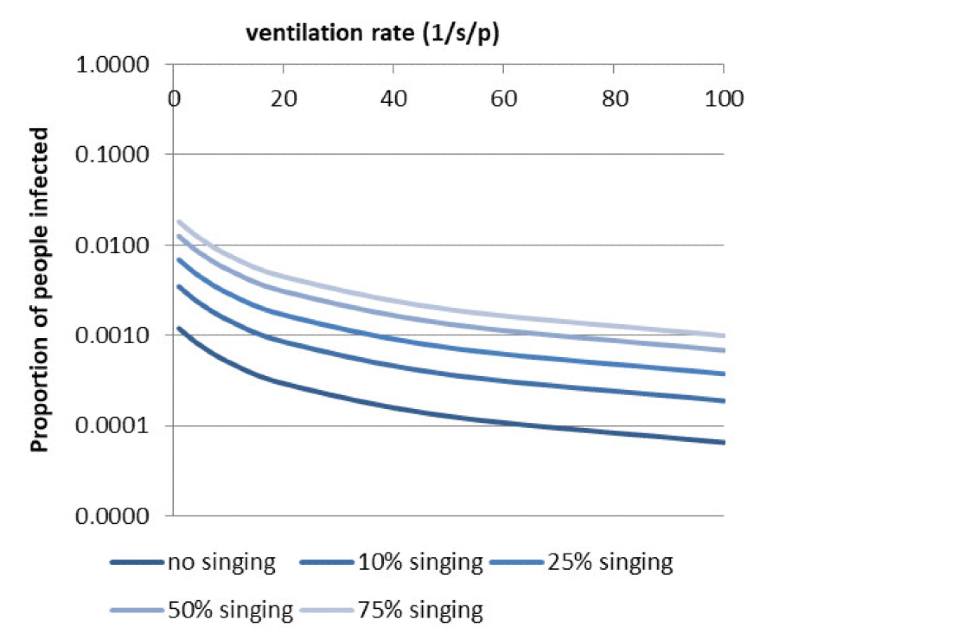
10 quanta per hour for a 60 minute event.
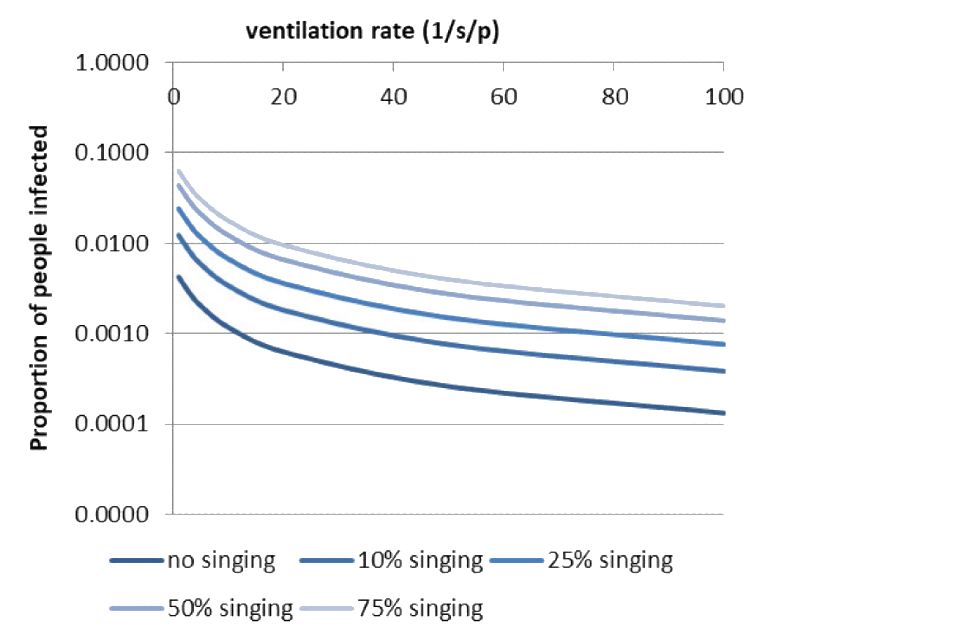
10 quanta per hour for a 120 minute event.
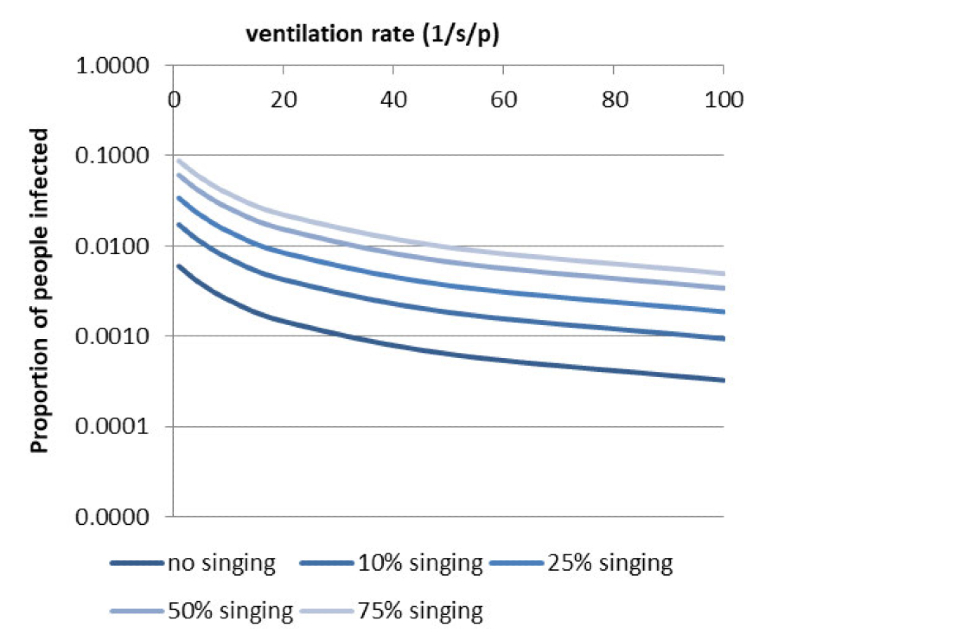
50 quanta per hour for a 60 minute event.
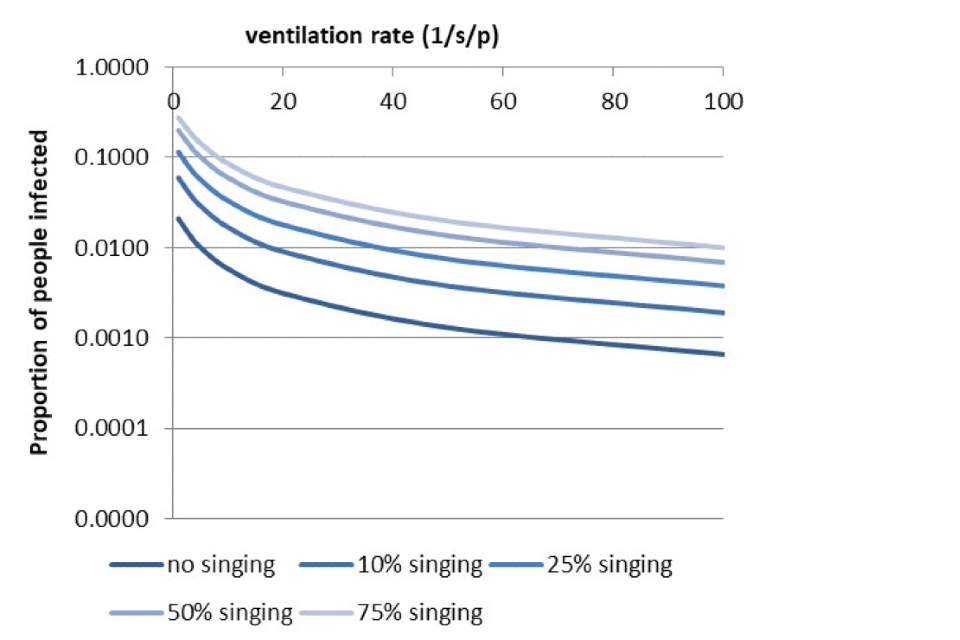
50 quanta per hour for a 120 minute event.
Figure 2: Proportion infection with ventilation rate and percentage of singing in a community venue
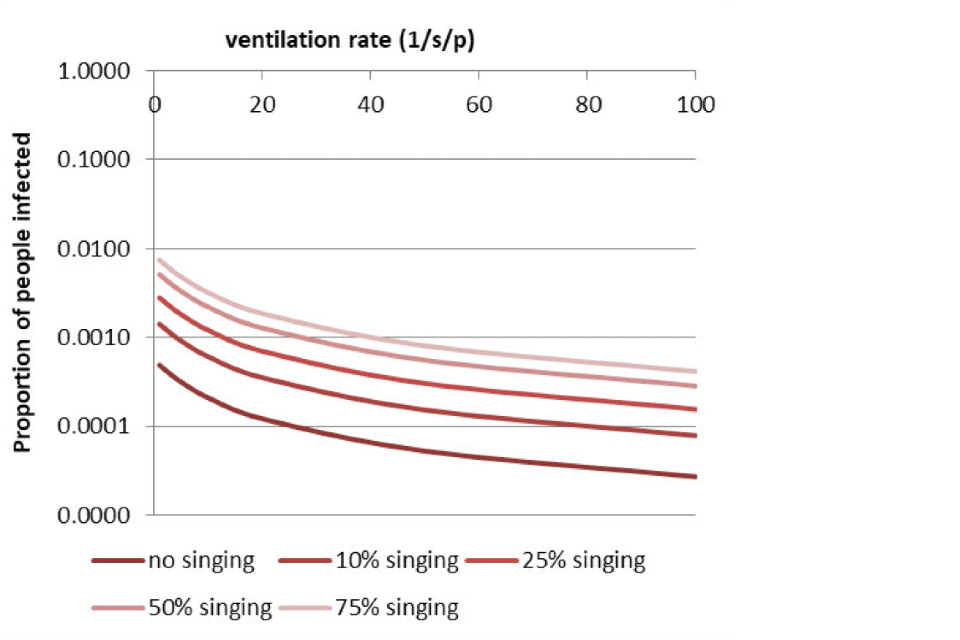
1 quanta per hour for a 60 minute event.
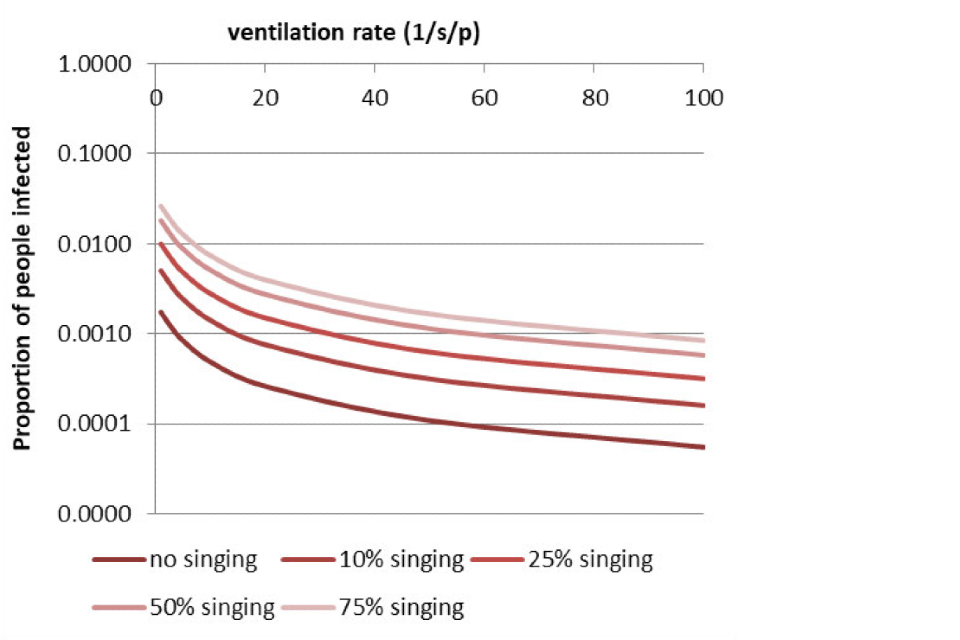
1 quanta per hour for a 120 minute event.
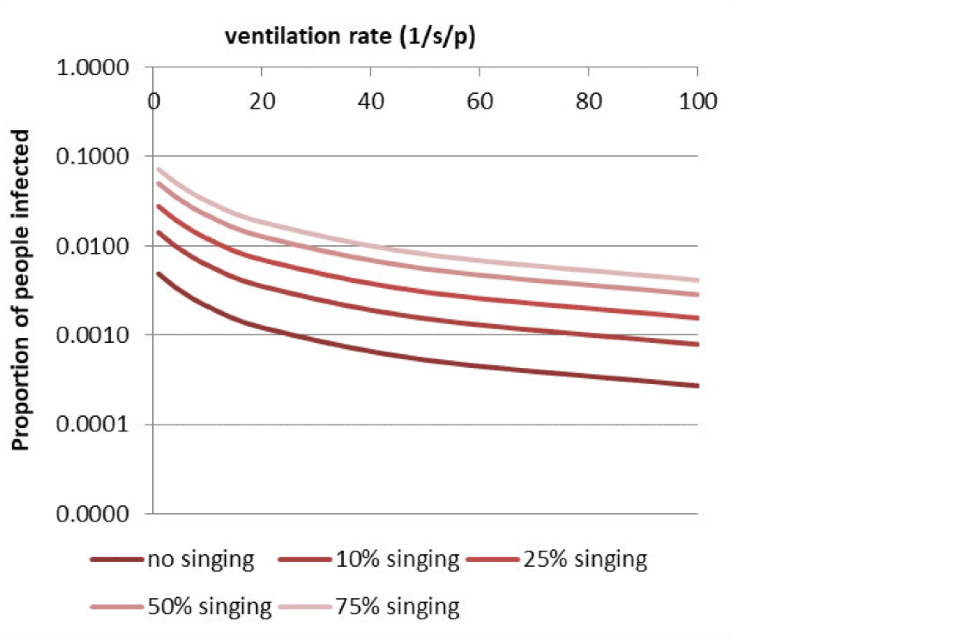
10 quanta per hour for a 60 minute event.
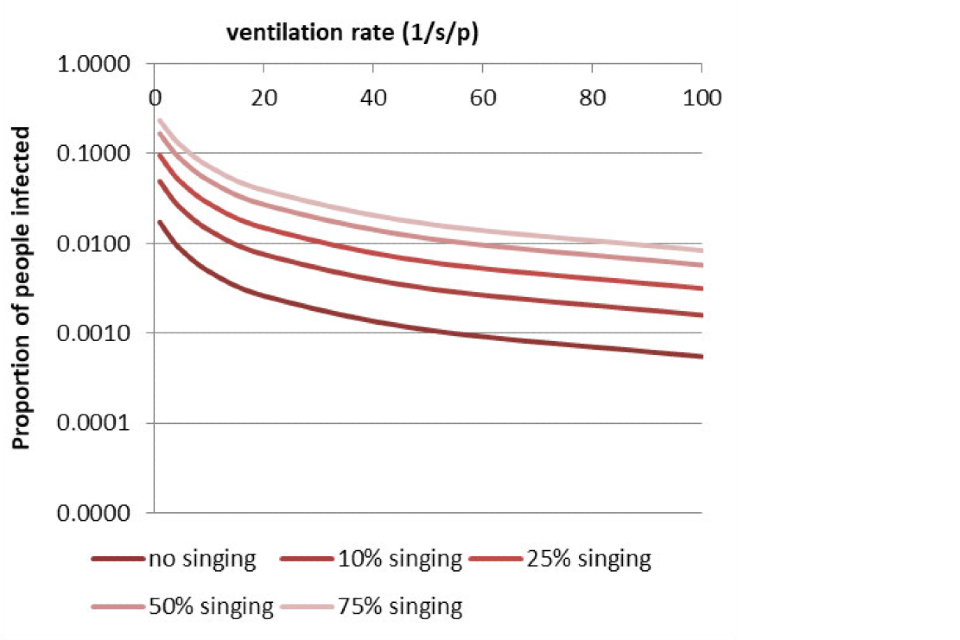
10 quanta per hour for a 120 minute event.
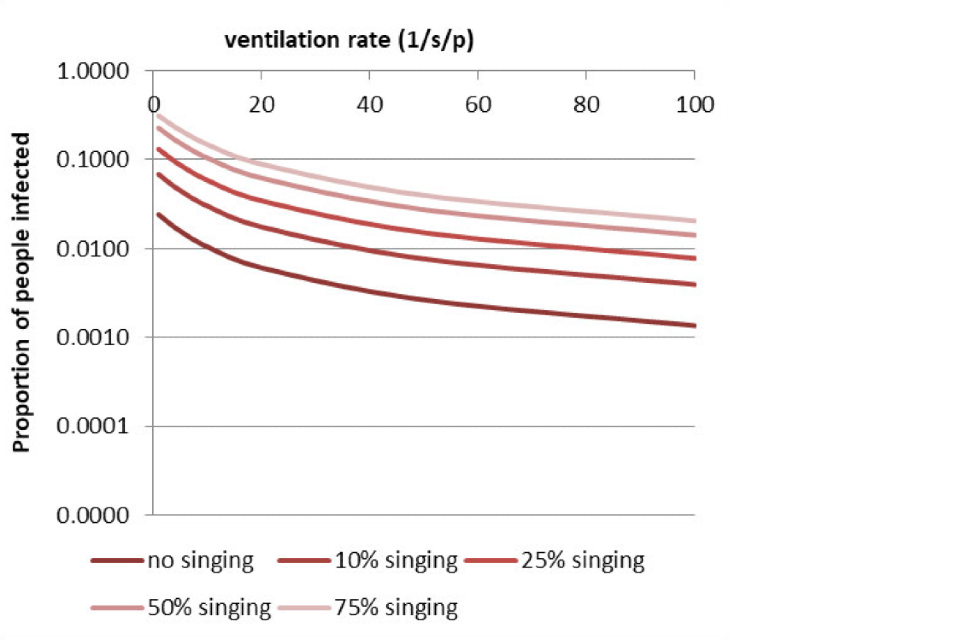
50 quanta per hour for a 60 minute event.
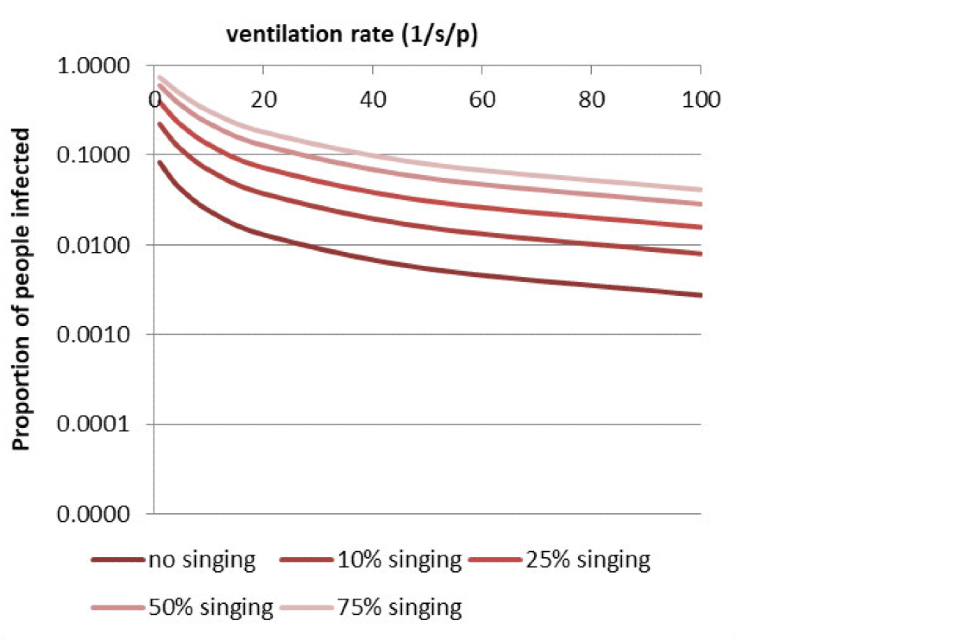
50 quanta per hour for a 120 minute event.